
Cancer, Recovery and Exercise

Figure 1. The role of genes and environment in the development of cancer [Adapted from Anand et al. 2008 [Link])
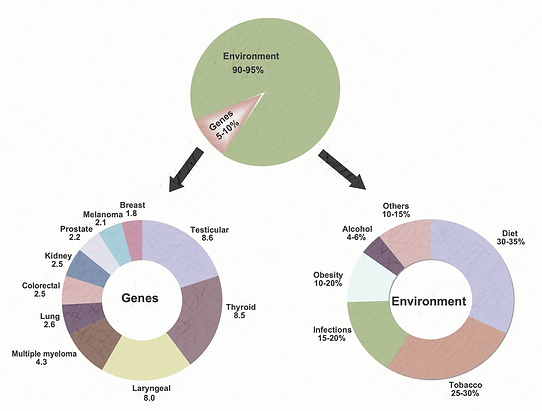
Introduction
​
One in two adults will be directly affected by cancer before the age of 75, with breast, prostate, and bowel cancers accounting for over half (53%) of all new cancer cases in the United Kingdom in 2017 (Cancer Research UK). In 2017, approximately 187,000 new cancer cases were reported in males and 179,000 new cases in females. This equates to approximately 367,00 new cancer cases in the United Kingdom each year. The older population group (>75 years or older) accounts for 36 per cent of all cancer cases. Regrettably, this indicates that every two minutes someone in the United Kingdom will be diagnosed with cancer.
​
Cancer continues to be a global killer, despite the high volume of research and pioneering work performed over the last decade. By 2020 the worldwide population will be approximately 7.5 billion and from this, it is projected that 15 million new cancer cases will be diagnosed, and regrettably, 12 million cancer patients will die (Brayand and Moller (2007). Cancer is caused by both internal and external factors and environmental issues (Figure 1). The association between physical activity, diet, and cancer is observed from varying rates of specific cancers in several countries. For example, Asians have been reported to have a 25 times lower occurrence of prostate cancer and ten times lower incidence of breast cancer than inhabitants of Western countries. However, when Asians migrate to the West the rates for these cancers increase substantially (Diet and Report [Link]).
​
​
​
​
​
​
​
​
​
​
​
​
​
​
​
While the particular causes of many cancers remain undetermined, lifestyle factors including physical activity levels are considered causal and modifiable for some. There have to date been over 190 reports from epidemiological studies and over 10 reviews examining the relationship between physical activity and cancer risk since the first reports in 1922 (Kruk & Aboul-Enein, 2006). These findings by Cherry (1922), and Sivertsen and Dahlstrom (1922) reported that mortality rates accounting for cancer in England, the United States, and Australia among men declined with increased work-related physical activity. Since these early studies, there has been a large body of evidence that has demonstrated that 80-to-90% of human cancer may be attributable to environmental and lifestyle-induced factors (i.e. dietary habits, physical activity, alcohol consumption, and tobacco use [McPherson et al., 1994; Brewster & Helzlsouer, 2001; Kushi & Giovannucci, 2002; Okasha et al., 2003, Murthy & Mathew 2004]). The scientific evidence supporting physical activity as a preventative measure is now plausible for certain cancers (colon/colorectal, breast, prostate, lung, and endometrial), with physical activity groups demonstrating a 25% risk reduction (Kruk & Aboul-Enein, 2006). However, evidence to date is limited for melanoma, testicular, ovarian, kidney, pancreatic, and thyroid cancers. This, therefore, makes it is difficult to draw any causal inferences on physical activity and cancer prevention.
​
There has been evidence on the importance of lifestyle factors and the development of certain cancers. Lichtenstein et al. (2000) studied the contribution of hereditary factors from data from 44,788 Swedish, Danish, and Finnish monozygotic twins. The authors found that at least one cancer occurred in 10,803 persons and among 9512 pairs of twins. Additionally, there was an elevated risk among the twins of affected persons for stomach, colorectal, lung, breast, and prostate cancer. There also were statistically significant effects of heritable factors for certain cancers including prostate (42%), colorectal (35%), and breast cancer (27%). Lichtenstein and colleagues concluded that the environment was an overriding contributor to the causation of cancer in the studied twins.
​
Pathology and Pathophysiology of Cancer
Animation 1. Tumour immunology and immunotherapy (Credit. Nature video [YouTube]).
Cancer is a major public health concern globally. The term cancer is a synonym for malignant neoplasm and has eight biological capabilities during the multistep development of human tumours. These capabilities include continued proliferative signalling, evading growth suppressors, resisting cell death, enabling replicative immortality, inducing angiogenesis, activating invasion and metastasis, reprogramming of energy metabolism, and eluding immune destruction (Hanahan and Weinberg, 2011). Unregulated cell growth without invasion is a feature of benign neoplasms.
We term cells that grow out of control and form a mass as a tumour or neoplasm (i.e., “new growth”). Some tumours are referred to as benign and develop specifically at the site where they began. There are also other tumours (i.e., malignant or cancerous) that can invade and destroy the normal tissue around them, and migrate throughout the body. It is important to understand that cancer is not a solitary disease, but rather a collection of several diseases. Cancer cells, however, share several distinct characteristics including changes in the genes that regulate cell division, programmed cell death and cell mobility. These genetic alterations lead to the characteristic features of cancer including the amassing of abnormal cells, invasion of adjacent tissues, and the migration to other distant sites.
Cancer, therefore, is the failure of controls over cellular birth and cellular death (Animation 1). Through eras of scientific inquiry, we have determined the design of cellular controls that project against tumours and the architecture of tissue restrictions that alter the progression of the disease. It is also important to understand that just because an individual has a specific set of genes and is exposed to certain environmental conditions does not mean we can determine when cancer will develop at a certain age or rate. According to the age-specific incidence curve, failure happens at varying rates and different ages. Therefore to understand cancer we must recognise the genetic and environmental factors that determine the incidence curve (Animation 2).
​
Animation 2. Tumour immunology and immunotherapy (Credit. Nature video [YouTube]).
Cancers are categorised into several groups, depending on the type of normal cell from which they developed. The most common forms of cancer develop from epithelial cells that line the body’s exteriors. We term these cancers as carcinomas, and they include breast, lung, cervical, prostate and colon cancers. Cancers can also develop from the cells of the blood (i.e., leukaemia), the immune system (i.e., lymphomas), and bone and connective tissues (i.e., sarcomas).
​
Armitage and Doll (1954) developed a multistage theory to analyse rates of cancer progression. The authors observed that cancer develops through a sequence of component failures leading to system failure. For example, each component failure, such as the abolition of a critical DNA repair pathway, move the entire system one stage along the progression if disease. Rates of component failure and the number of stages in progression determine the age-specific incidence curve. Mutations that increase the rate of transition between stages or knock-out a component shift the incidence curve to earlier ages (Figure 1).
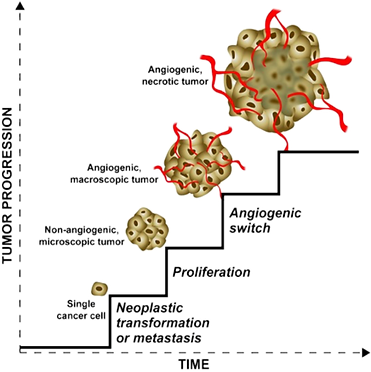
Figure 1. The main phases of tumour progression. Image adapted from Naumov, Folkman and Straume (2009).
At the initial onset, there is a single cancer cell. This cell may originate from normal cells through neoplastic transformation (a complex process by which a normal cell becomes a tumour cell). Alternatively, this cell might be a metastasis (i.e. a cell that migrates from an already established tumour). The single cancer cell proliferates and generates a population of cells that form a microscopic solid tumour. The tumour is small and cells can take up nutrients directly from the adjoining environment. The small tumour stimulates the formation of blood vessels (a process is called the angiogenic switch). The newly formed blood vessels migrate in the tumour and deliver nutrients to tumour cells. Without blood vessels a solid tumour can grow up to the size of ~1 mm3 and then stops growing because of nutrient restriction; with new blood vessels, the tumour starts growing again. The growth is unlimited and the tumour volume increases, the network of blood vessels, is not organised. Blood vessels can not deliver nutrients to all cells in the tumour and many cells die forming large areas of necrosis. All these steps involve a significant number of different genes and molecular paths to take place.
​
Mutations of Cells
​
Mutations are not only a trademark of cancer but may be essential in cancer progression. Cancer cells typically divide where normal cells do not; they annex, metastasise and eradicate the host of origin. The available evidence suggests that cancer is heritable at the cellular level and that cancer cells comprise of various mutations, signifying that tumour progression is determined by mutagenesis.
Molecular procedures over the last decade have made it easier to examine the human genome, from analysing chromosomes down to genes and even nucleotide sequences. With deeper levels of investigation, more mutations are being recognised in cancer cells. The evolving hypothesis is that genomes of cancer cells are unstable, and this variability results in a cascade of mutations which in some cases enable cancer cells to circumvent the host regulatory processes that govern cell location, division, expression, adaptation and death. Within each tumour genetic instability is displayed by extensive heterogeneity of cancer cells. Moreover, tumours continually develop resistance to chemotherapeutic agents. Each of the tumour phenotypes involves, or can be replicated by, certain mutations introduced in essential genes. These mutations either develop from replicating unrepaired DNA damage or from errors occurring during DNA synthesis.
Mutations as a result of DNA damage
​
It is commonly accepted that, rather than being inactive, cellular DNA undergoes a continual process of damage and resynthesis. DNA is damaged by environmental and endogenous (cellular) sources. Many of the environmental agents that damage DNA have been reported to be mutagens. Epidemiological evidence indicates that several of these agents are also human carcinogens. The relationship between environmental compounds and human cancers have been well documented in conditions where small groups of individuals have been exposed to an inordinately high concentration of a specific chemical that caused an uncommon tumour.
DNA damage by chemical compounds can be separated into two groups: (1) those that produce large adducts and are repaired by the nucleotide excision pathway; and (2) those that cause minor alterations and are repaired by the base excision repair pathway (Loeb and Loeb, 2000). Ames et al. (1995) suggest that in addition to DNA damage caused by environmental agents, many foods contain natural chemicals that can also damage DNA and produce comparable alterations. Ames and colleagues (1990) argued that consuming food with natural DNA-damaging chemicals poses a greater risk than chemicals produced from industry. Another feasible mechanism that potentially damages the DNA is the cellular metabolic processes which produce reactive chemical intermediates feasibly causing spontaneous mutations and cancers (Loeb, 1989). Furthermore, Lindahl and Nyberg (1972) provided evidence that suggested water may even possibly damage DNA, triggering hydrolysis of the glycosylic bond and the development of mutagenic abasic sites in DNA. This was centred on rates of depurination of DNA in vitro, where it was estimated that each cell undergoes 10 000 depurination per day.
Cellular mechanisms have evolved to repair each DNA lesion, but reflecting the frequency at which they occur and the compacted and esoteric structure of human chromatin, it is likely that a significant portion of these lesions escape DNA repair and produce mutations during replication of the unrepaired DNA by DNA polymerases. DNA polymerases, in general, duplicate past small variations (i.e. methyl or ethyl groups) with efficacy, and typically produce mutations depending on the miscoding potential of the transformed nucleotide (Loeb and Cheng, 1990). Large adducts are difficult to bypass by normal cellular polymerases and mutagenesis is reliant on the production of damage-inducible error-prone pathways involving special error-prone DNA synthesis (Murli and Walker, 1993). Combined, these results indicate that the nucleotide sequence of cellular DNA is maintained at a homeostatic equilibrium, such that an increase in the production of DNA damage or a reduction in DNA repair results in an increased frequency of mutations (Figure 4).
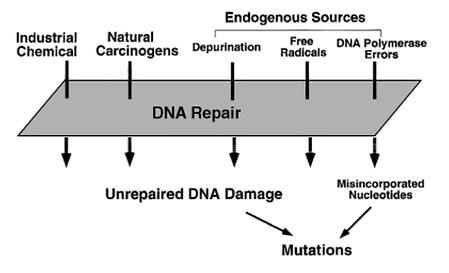
Figure 4. Aspects regulating mutation accumulation in cancer cells. A range of agents has the potential to damage DNA. Mutations are created by DNA damage that evades the various mechanisms for DNA repair. Consequently, there is a dynamic equilibrium between DNA damage and DNA repair and this equilibrium is disturbed in cancer cells.
Risk Factors of Cancer
Cancer is caused by an array of identified and unidentified factors. The most significant recognised cause of cancer is tobacco smoking. Other important causal factors of cancer risk include diet, alcohol and physical activity, infections, hormonal factors and radiation. The relative importance of cancers as a cause of death is increasing, typically because of the increasing proportion of individuals who are old, and also because of reductions in mortality from some other causes (i.e. infectious diseases). The prevalence of cancers of the lung, colon and rectum, breast and prostate mostly increase in parallel with economic development, while the incidence of stomach cancer declining with economic development.
Cancer is a major cause of mortality worldwide and, in the developed world, is exceeded only by cardiovascular diseases. It has been estimated that 10 million new cases and over 6 million deaths from cancer occurred in 2000 (Parkin, 2001). As developing countries become urbanised, patterns of cancer, including those strongly allied with diet, tend to shift towards economically developed countries. Between 2000 and 2020, the total number of cases of cancer in developing has increased by over 70% and, in the developed world, to increase by over 25%, mainly as a result of an increase in the number of old people.
Smoking Tobacco

In 1964 the Surgeon Generals Advisory Commission Report identified that smoking was a primary cause of lung cancer and efforts have been made to reduce usage. This landmark report was the first that associated smoking cigarettes with dangerous health effects including lung and laryngeal cancer in males and a probable cause of lung cancer in females (Alberg, Shopland, and Cummings, 2014 [Link]). The carcinogenic effects of smoking are well documents, for example in 1993 the United States Environmental Protection Agency categorised environmental tobacco smoke as a human lung carcinogen. Tobacco contains over 50 carcinogens with several associated with lung cancer. Among the many components of tobacco smoking, polycyclic aromatic hydrocarbons are strongly implicated as causative agents in the development of cancer (Hecht, Carmella and Murphy, 1993). For example, one tobacco metabolite benzopyrenediolepoxide are implicated in the development of lung cancer (Denissenko et al. 1996 [Link]).
Fortunately, among developed countries, tobacco smoking has been slowly declining, unfortunately in developing counties the prevalence of smoking is growing. Recent data has suggested that 71% of the worlds tobacco usage will be from developing countries especially East Asia ( Anand et al. 2008). Government driven control programs in areas of increased usage are now implemented in which to reduce the rates of tobacco-related cancer mortality.
There is at present limited evidence that demonstrates fully how smoking tobacco contributes to various cancers. However, the evidence suggests that tobacco smoking alters several cell signalling pathways with a strong association between tobacco smoking and inflammation. A study by Shishodiaaan and Aggarwal (2004) reported that tobacco smoke can induce activation of nuclear factor-kB (NF-kB), an inflammatory marker. Therefore, anti-inflammatory agents that can suppress NF-κB activation may be used against cigarette smoke. Further evidence from Anto et al. (2002) demonstrated that a dietary agent (curcumin) can inhibit the NF-κB induced by cigarette smoke. There is some evidence that shows that several dietary agents reduce the carcinogenic effects of tobacco smoke.

Figure 5. Cancers have been associated with alcohol and smoking. Percentages represent the cancer mortality attributable to alcohol and smoking in men and women [Adapted from Anand et al. 2008).
Alcohol Consumption
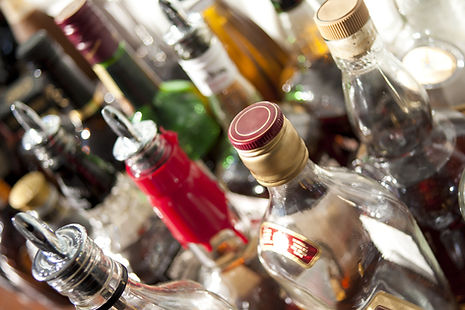
As far back as 1910, there has been a known association between alcohol and cancer (Tuyns, 1979). Since then there have been several studies that have reported the association between chronic alcohol consumption and cancers of the oral cavity, pharynx, oesophagus, liver, colon, rectum and in women breast cancer (Maier et al. 1994; Seitx, Stickel and Homann, 2004). In the upper aerodigestive tract, 25-to-68 per cent of cancers are attributable to alcohol, and up to 80% of these tumours can be prevented by abstaining from alcohol and smoking (La Vecchia et al. 1997).
Seitz et al. (2012) reported that there is a significant risk by four per cent if women intake one alcoholic drink per day. Heavy alcohol consumption (three or more days per week) is associated with an increased risk of 40-to-50 per cent. The authors attributed up to five per cent of breast cancers to alcohol consumption. This is further supported by Hamajima et al. (2002) who reanalysed epidemiological studies on hormonal factors in women and the association between alcohol and breast cancer. The authors reported a 7.1% increase in relative risk of breast cancer for each additional 10 g/day of alcohol consumed. In addition to it being a risk factor for breast cancer, heavy intake of alcohol (more than 50–70 g/day) is a recognised risk factor for liver and colorectal cancers.
Furthermore, there is a body of evidence relating to heavy alcohol consumption and hepatitis C virus or hepatitis B virus, which increases the risk of hepatocellular carcinoma by dynamically stimulating cirrhosis. The relationship between alcohol and inflammation has also been well established, especially in terms of alcohol-induced inflammation of the liver. However, how alcohol contributes to carcinogenesis is not clearly evidenced but research suggests that ethanol may play a significant role. Poschl and Seitx (2004) suggest that ethanol is a cocarcinogen rather than a carcinogen especially when ethanol is metabolised. Ethanol is metabolised acetaldehyde and free radicals are produced, with free radicals believed to be associated with alcohol carcinogenesis via binding to DNA and proteins.
Alcohol stimulates carcinogenesis by other mechanisms including the induction of cytochrome P-4502E1. This cytochrome is associated with the enhanced production of free radicals and enhanced activation of several procarcinogens that are contained in alcoholic drinks. This leads to a change in metabolism and in the distribution of carcinogens, in association with tobacco smoke and diet. These alterations due to individuals lifestyle, change the behaviour in cell-cycle duration leading to hyperproliferation, nutritional deficiencies ( e.g. methyl, vitamin E, folate, pyridoxal phosphate, zinc, and selenium) and changes of to the immune system.
Alcohol also increases the occurrence of tissue injury (i.e. cirrhosis of the liver) and can activate the NF-κB proinflammatory pathway (Szabo et al. 2007) and can contribute to tumorigenesis ( Aggarwal, 2004 [Link]). Evidence also demonstrates that benzo[a]pyrene can infiltrate the oesophagus when combined with ethanol (Kuratsune et al. 1965). Therefore anti-inflammatory agents may be effective for the treatment of alcohol-induced toxicity.
Dietary Consumption
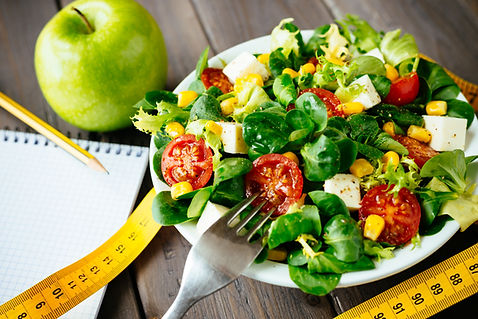
The World Health Organisation has estimated that approximately 30-35% per cent of cancers are attributed to dietary factors. Dietary factors are second only to tobacco as a hypothetically preventable cause of cancer. Cancer rates vary as populations migrate between countries and adopt different dietary customs, further implicating dietary factors in the aetiology of cancer. The association between body composition and physical inactivity account for approximately 20-to-30 % of the most common cancers (including colon, endometrium, kidney, breast and oesophagus).
Research to date has not fully established the relationship between diet and cancer risk. Evidence associated with dietary factors for an increase in risk are overweight and obesity, and high consumption of alcoholic beverages, aflatoxins, and some forms of salting and fermenting fish. There is also other evidence to indicate that physical activity decreases the risk of colon cancer. After tobacco, overweight and obesity appear to be the most important known avoidable causes of cancer.
Most carcinogens that are consumed, such as nitrates, nitrosamines, pesticides, and dioxins, come from food or food additives from cooking. High dietary consumption of red meat is a risk factor for several cancers, especially for those of the gastrointestinal tract, but also for colorectal (Bingham, Hughes and Cross, 2002; Chao et al. 2005), prostate (Rodriguez et al. 2006), bladder (Garcia-Closas et al. 2007), breast (Tappel, 2007), gastric (O’Hanlon), pancreatic, and oral (Toporcov, Antunes and Tavares, 2006) cancers. This is in contrast to a study by Dosil-Diaz et al. (2007) who reported that meat consumption reduced the risk of lung cancer.
It is commonly considered that ingestion of red meat increases the risk of cancer due to the heterocyclic amines produced during cooking being carcinogenetic. The frequent cooking with charcoal and smoke curing of meat creates harmful carbon compounds including pyrolysates and amino acids, which have a cancerous effect. For example, Lauber and Gooderham (2007 [Link]) investigated cooked meat-derived heterocyclic amine 2-amino-3-methylimidazo[4,5-b]pyridine (PhIP) and mutations. They observed that PhIP at doses as low as 10(-11) mol/L has direct effects on a rat pituitary lactotroph model and can induce cell proliferation and the synthesis and secretion of prolactin. The authors concluded that there is a strong association between estrogen, progesterone, prolactin, and breast cancer. The PhIP range of hormone-like activities provides mechanistic support for the tissue-specific carcinogenicity of the chemical. Moreover, recent epidemiology studies report an association between consumption of cooked red meat and premenopausal and postmenopausal human breast cancer. The PhIP is the most prevalent mutagen by quantity in cooked beef and is accountable for ~20% of the total mutagenicity found in fried beef. The daily intake of PhIP among Americans is estimated to be 280–460 ng/day per person (Lauber and Gooderham, 2007).
Nitrites and nitrates preservatives that are often used in meat as they bind to myoglobin, preventing botulinum exotoxin production however, they are also strong carcinogens (Divisi, Tommaso and Savemini, 2006). Sasaki et al. (2002) observed that long-term exposure to these nitrite preservatives and azo dyes have been associated with the induction of carcinogenesis. Moreover, bisphenol from plastic food containers can migrate into food and may increase the risk of breast (Durando et al. 2007) and prostate (Ho et al. 2006) cancers. Szymanska-Chabowska, (2002) provided evidence on the ingesting of arsenic-contaminated drinking water and the increased risk of bladder, kidney, liver, and lung cancers. Saturated fatty acids, trans-fatty acids, and refined sugars and flour present in most foods have also been associated with various cancers. Several food carcinogens have been shown to activate inflammatory pathways.
Obesity and Cancer

It has been estimated that about 20% of all cancers are produced by excess weight (Wolin, Carson and Colditz, 2010). The Million Women Study has also observed that approximately half can be attributed to obesity in postmenopausal women (Reeves et al. 2007). Several epidemiological studies have demonstrated a direct association between overweight and cancer, even though obesity alone does not heighten cancer risk in all tissues by the same amount [Calle and Kaaks, 2004)].
Renehan et al. (2008) performed a meta-analysis of 221 datasets including 282,137 incident cancer cases. The authors reported that in men an increase in BMI by 5 kg/m2 was strongly associated with oesophageal adenocarcinoma (Risk Ratio 1·52, p<0·0001) and with thyroid (1·33, p=0·02), colon (1·24, p<0·0001), and renal (1·24, p <0·0001) cancers. In women, a strong association between a 5 kg/m2 increase in BMI and endometrial (1·59, p<0·0001), gallbladder (1·59, p=0.04), oesophageal adenocarcinoma (1·51, p<0·0001), and renal (1·34, p<0·0001) cancers. With weaker positive associations between BMI and rectal cancer and malignant myeloma, and non-Hodgkin lymphoma in both male and females. However, associations between ethnic groups reported that that African Americans appear susceptible to cancer, in contrast to Hispanics who appear to be somewhat protected while the association of increased BMI with breast cancer is strong in Asia-Pacific populations.
Obesity is a severe problem that increases the risk of several chronic illnesses including cancer development (Renehan et al. 2008). Current recommendations from the Public Health Goals of the World Cancer Research Fund recommend that the average adults BMI should be maintained between 21 and 23 kg/m2, depending on the normal range for different populations (World Cancer Research Fund, 2007). Obesity plays a central role in carcinogenesis and has been brought to the forefront by data specifying the rapid increase of oesophagus adenocarcinoma over the past two decades.
Furthermore, evidence on weight accumulation with age is also related to an increase in postmenopausal breast cancer risk in women who do not comply with a menopausal hormone therapy routine (Ahn et al. 2007)]. A study by Hankinson et al. (1995) reported that breast cancer risk was lowered by 50% in women who purposely underwent weight loss greater than 10 kg after menopause. Moreover, the Swedish Obese Subjects (SOS) study, established that bariatric surgery achieves an average of 20 kg weight reduction in obese patients with a BMI higher than 40 kg/m2. This weight loss corresponded to the surgery group who had untreated morbidly obese women and reported a significant reduction in cancer incidence in association with weight loss on a follow-up longer than 10 years (Sjostrom et al. 2009).
Regarding the role of childhood obesity in adult cancer, a study performed by Jeffreys et al. (2004) assessed cancer risk related to age and sex-specific BMI standard deviation scores (SDS) of subjects aged 2-to-14. Among these subjects, the risk of cancer in adult life was increased by 9% per SD increase in childhood BMI. Furthermore, an excess of weight in teenagers was associated with doubling the mortality risk of colon cancer in adulthood (Bjorge et al. 2008). Studies have shown that the common denominators between obesity and cancer include neurochemicals; hormones such as insulin-like growth factor 1, insulin, leptin; sex steroids; adiposity; insulin resistance; and inflammation (Hursting et al. 2007). The involvement of signalling pathways including the IGF/ insulin/Akt signalling pathway, the leptin/JAK/STAT pathway, and other inflammatory cascades have also been associated with obesity and cancer (Hursting et al. 2007). For example, hyperglycemia has been shown to activate NF-κB (Nareika et al. 2008), which could link obesity with cancer. Also known to activate NF-κB are several cytokines produced by adipocytes, such as leptin, tumour necrosis factor, and interleukin-1 (Tang et al. 2007). Energy balance and carcinogenesis have been closely connected (Hursting et al. 2007). However, whether inhibitors of these signalling cascades can reduce obesity-related cancer risk remains unanswered.
Treatment and Management of Individuals with Cancer

As discussed above cancer is not one disease and as a result, the signs and symptoms of cancer are not universal but cancer-specific. That said, most signs and symptoms are similar to those of other medical conditions and, therefore, their occurrence is not necessarily symptomatic of cancer. However, when these symptoms occur individuals must have them assessed by a physician or doctor. Table 1 summarises the main signs and symptoms for the most common cancer sites.
Table 1. Signs and Symptoms of Several Common Cancers.
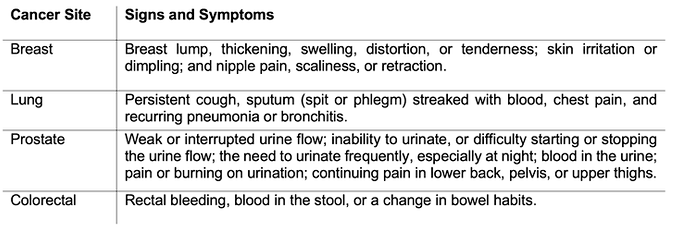
The treatment and management of individuals with cancer are dependent on the type and stage of cancer. The type and stage must be determined when selecting the most effective mode of treatment. The American Cancer Society (2016) uses the TNM (Tumour, Node, Metastasis) system that provides essential information and is the most frequent method applied:
​
-
T: Denotes the size of the tumour and is graded as T0 (no tumour) to T4 (tumour invasion of a vital organ such as the lungs).
-
N: Denotes whether the lymph nodes are affected by cancer; this factor is scored as N0 (no invasion of the lymph nodes) to N3.
-
M: Denotes the presence or absence of cancer metastasis to other organs of the body and is termed M0 (no metastasis) or M1 (metastases present).
​
For instance, a tumour categorised as T1N0M0 signifies a small tumour that has not migrated to the lymph nodes or metastasised. When TMN has been determined and the tumour defined, they are pooled together in an inclusive stage grouping including a set of stages from stage 0 to IV. For example, the lower the stage number (i.e., stage 1) are used to describe a small primary tumour and no metastases. Whereas the higher numbers (i.e., stage IV) describe cancer that has metastasised to other areas throughout the body.
After an individual has received treatment for cancer, they may undergo a period of remission lasting several weeks to years. During this period, the cancer is responding to the subsequent treatment or is under control. For cancer to be categorised as being in complete remission, there has to be clear evidence that all signs and symptoms of the disease have disappeared. However, some individuals may be in partial remission with signs of improvement in symptoms, but these do not entirely disappear. If there is a relapse or recurrence in cancer, then further treatment may be required, which often leads to another period of remission.
​
Currently, there are four types of treatment for cancer, namely surgery, radiation, chemotherapy and a range of biological therapies. Treatment and therapy may be combined to treat the disease. Locally the use of radiation therapy is the primary mode of treatment and focuses on the tumour but has limited effect elsewhere in the body. This therapy delivers high-energy particles (e.g., x-rays or gamma rays) that destroy or damage the cancer cells. This treatment breaks apart the DNA molecule inside the cell, therefore, preventing the growth and division of the cancer cell. Radiotherapy therapy can be delivered in two ways:
​
-
Internal radiation or brachytherapy. This type of radiation is delivered either by a small radioactive container being positioned inside the tumour or in the area of the incision after surgery, destroying or damaging the tumour cells. Another approach involves the administration of radioactive drugs (radiopharmaceuticals) by mouth or by injection.
​​​​
-
External radiation. This treatment involves specialist machinery that delivers a high-energy particle to the cancer site and adjacent tissue. This treatment is usually given five days a week for five to eight weeks. However, modern technology including intensity-modulated radiation therapy [IMRT]) and three-dimensional conformal radiation therapy (3D- CRT) induce less harm to adjacent tissues and provide higher dosages to the tumour.
​
Chemotherapy. This treatment involves the administration of chemotherapeutic drugs into the bloodstream to treat cancer cells that have metastasised to other regions of the body. This treatment can last from several months to years and is given cyclically followed by a period of recovery. However, there are several considerations regarding the duration of chemotherapy treatment including the type of tumour and phase, individual’s tolerance to chemotherapy and their general physical well-being. This mode of treatment may significantly reduce the risk of surgery and cancer recurrence. These drugs have several modes of action including the disruption of DNA replication and disruption of normal cellular architecture necessary for cell structure and shape. Recent advancements in chemotherapeutic drugs now target specific enzymatic functions in cancer cells that regulate growth (termed “targeted” therapies).
​
Biological therapy. This form of treatment also includes immunotherapy, biotherapy, or biological response modifier therapy and uses a variety of large (typically protein) molecules to fight cancer or to reduce the side effects of specific cancer treatments. This type of therapy inhibits cancer cell growth and aids the healthy immune cells to control cancer in specific treatments or aid in the repair of normal cells damaged by other types of cancer treatment.
​
Bone marrow transplantation. This therapy is used to treat blood cancers including leukaemia and Hodgkin lymphoma. In this form of treatment, the diseased bone marrow is destroyed using large doses of chemotherapeutic agents, radiation, or a combination. The individual’s blood-forming system is then exchanged with either bone marrow cells from the individual when they are in remission (autologous bone marrow transplant) or with a tissue antigen-matched donor (allogeneic).
​
Hormonal therapy. This treatment uses drugs that modify the body's hormones intending to obstruct the growth of the tumour. Several tumours have hormonal receptors in their cells, and their growth depends on hormones. These forms of tumours are called hormone receptor-positive and can be treated with hormone therapy. In cancers that are hormone receptor-negative, this kind of treatment is not beneficial. The tumours that are hormone receptor-positive include prostate and breast cancer:
​
-
Breast cancer: Almost 66% of breast cancers are hormone receptor-positive. The hormone estrogen increases tumour growth of breast cancers that are hormone receptor-positive. These tumours have hormone receptors for estrogen (ER-positive cancers), progesterone (PR-positive cancers) or both. The aim of hormone therapy for breast cancer is to reduce estrogen levels.
​
-
Prostate cancer: The male hormones (androgen hormones) increase the tumour growth of prostate cancer. The aim of hormone therapy for prostate cancer is to reduce testosterone levels (e.g., androgen). The hormone therapy for prostate cancer is also termed androgen deprivation therapy.
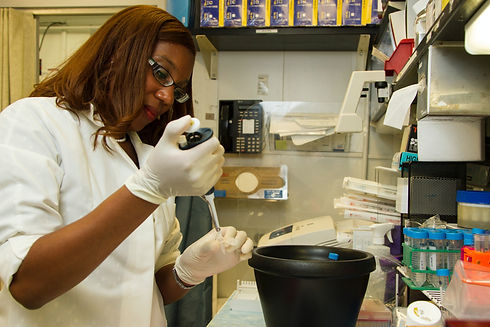
Side Effects of Cancer Treatment
​
When individuals undergo cancer treatment there is ultimately disruption in their body functioning which may also affect healthy cells and tissues. The side effects severity is dependent on the type of drug or radiation, the dosage given and the duration and length of treatment (Table 2 and 3). The initial side effects that patients may experience include nausea and vomiting, increased risk of infections, pain, loss of appetite, hair loss and potential haemorrhagic and thromboembolic complications. The side effects also occur in the late stages of treatment and can develop over several months or years with some effects permanent. The most severe side effects of treatment include the toxic effects on numerous body systems and organs. These effects impair health-related quality of life and include hematologic and immune system toxicity; cardiovascular toxicity; pulmonary toxicity; musculoskeletal alterations; gastrointestinal system toxicity; endocrine toxicity; hepatic toxicity; nephrotoxicity and neural toxicity.
Table 2. Adverse Effects of Radiation Therapy.
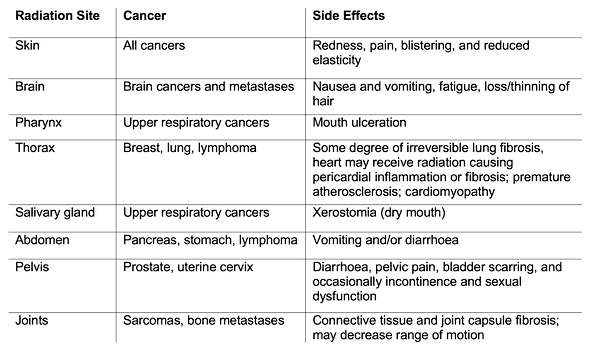
Table 3. Adverse Effects of Systemic Therapy.
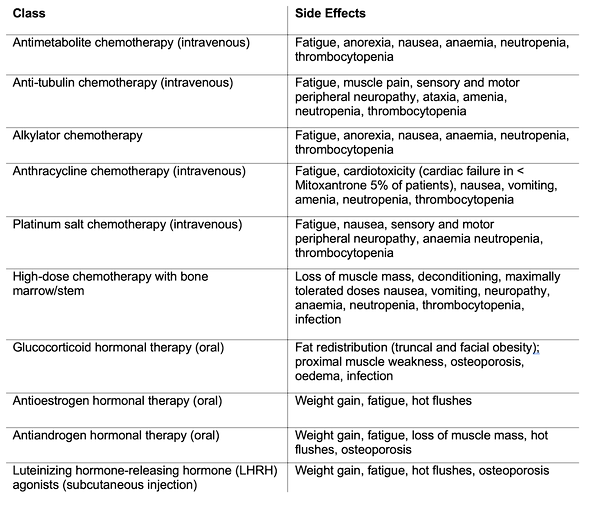
The physical effects of cancer treatment can lead to diminished cardiovascular and pulmonary function, muscle atrophy, sleep impairment, nausea, vomiting, pain and fatigue (Schmitz et al., 2005). These side effects generally peak during treatment, however, it has been recognised that cancer treatments can also result in long-term chronic effects and late effects (Aziz, 2007). Table 4 includes some of the common late effects of cancer treatment. The available body of evidence suggests that the health-related fitness components may be compromised at some period after a cancer diagnosis.
​
The effects of cancer treatment and reduced physical activity may result in the individual’s cardiorespiratory system and the musculoskeletal system is impaired and deconditioning (Schmitz et al., 2005; Aziz, 2007). Treatment of cancer can also result in negative changes to body composition including increased fat mass, decreased muscle mass (i.e., cachexia) and decreased bone density. Rooney and Wald (2007) reported that between 50-96% of female breast cancer patients experienced weight gain following diagnosis with an increase in fat mass and a decrease in muscle mass. This is supported by a two-site study by Segal et al., (2003) who reported [in part] that men diagnosed with prostate cancer and undergoing androgen deprivation therapy gained fat mass while losing lean muscle mass. Cancer survivors are also susceptible to bone mineral density loss caused by cancer and also the treatment (Guise, 2006). McNeely and associates (2006) stated that the quality of the bone can be affected. This is especially true with cancers that involve bone and hematologic malignancies. Certain treatments have been associated with bone loss including androgen deprivation therapy, certain chemotherapies, selective estrogen-receptor modulators, aromatase inhibitors, glucocorticoids, stem cell transplantation, and radiation treatment. Unfortunately, this reduction in bone minerals may lead to functional impairments and limitations including osteoporosis, resulting in an increased risk of fracture and restricted recovery from fractures (McNeely et al., 2006).
Table 4. Adverse Late Effects of Radiotherapy and Chemotherapy.
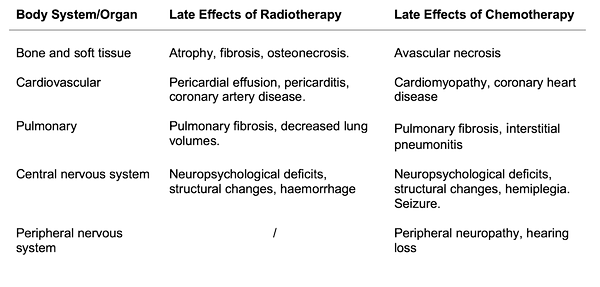
During cancer treatments, muscle weakness and atrophy occur which affects an individual’s strength and endurance. Studies have reported that the causes of lean mass loss can include neuropathy, cachexia, steroid treatment and physical deconditioning due to inactivity (Ardies, 2002; Schmitz et al., 2005; McNeely et al., 2006). Treatment including radiation and surgery can affect flexibility as an outcome of soft tissue damage, nerve damage, fibrosis or pain. This can result in the impaired or restricted function of the specific joint or area of the body undergoing treatment. For example, impaired shoulder function is often reported after mastectomy and axillary dissection and radiation for breast cancer (Blomqvist et al., 2004) and is a complication of neck dissection procedures (McNeely et al., 2004).
Cancer-Related Fatigue
The most prevalent reported side effect of cancer and cancer treatment is fatigue. Cancer-related fatigue is different from fatigue occurring from everyday activities and has been reported to affect almost 70% of cancer patients during chemotherapy and radiotherapy and after surgery (Dimeo, 2001). Cancer patients are advised to recover and down-regulate their activity levels. However, rest does not improve cancer-related fatigue as inactivity aids muscle catabolism and extended rest periods may lead to chronic fatigue (Dimeo, 2001; Lucia, Ernest and Perez, 2003). Research by Lucia and associates (2003) have reported that 30-50% of cancer survivors describe their fatigue levels after the treatment ends last for months.
​
The American Cancer Society (2016) has defined cancer-related fatigue as feeling tired (physically, mentally, and emotionally). Cancer-related fatigue has been characterised as being the most distressing side effect of cancer and its treatment. This may be due to the effects on the individual’s quality of life (Lucia, Ernest and Perez, 2003) and can be caused by cancer or cancer treatment lasting for an extended period. This leads to the patient’s daily activities difficult, and as the treatment continues so does the severity of the cancer-related fatigue.
​
The exact causes of cancer-related fatigue are not fully understood and have been reported to be multifactorial. For instance, anaemia, pain, depression and anxiety, sleep disturbances, nutritional issues, low levels of physical activity, medicines, and other medical problems may produce fatigue. Many patients describe fatigue as a physical disturbance with a loss of functionality during daily tasks such as walking, climbing, or completing basic household chores. Importantly, activity-limiting fatigue is caused by severe muscular deconditioning related both to illness and treatment, in addition to the inactive behaviour of the individual (Lucia, Ernest and Perez, 2003).
Cancer-related fatigue is predominantly due to the illness, the subsequent treatment and physical inactivity that has resulted in deconditioning. Unfortunately, the inactive lifestyle promoted by biomedical professionals and family members may lead to the development of a self-perpetuating fatigue cycle (Figure 1.). The American Cancer Society (2016) recommends physical activity as a component within the treatment to break the cycle of self-perpetuating fatigue and to combat fatigue.
Figure 1. Sedentary habits can transform cancer-related fatigue into chronic fatigue.

Lymphedema
​
Lymphedema may occur in cancer patients, especially after radiotherapy or after surgical removal of lymph nodes (lymphadenectomy). These procedures produce a partial obstruction of the lymphatic system due to the lower number of lymph nodes or a reduced lymph flow or both. These atypical conditions typically result in interstitial fluid build-up and cause swelling and inflammation due to lymph fluid accumulation usually caused by obstructions of the lymph system in the extremities.
Shaw and colleagues (2007) reported that lymphedema may occur within a few days and even up to 30 years after breast cancer treatment. Petrek et al., (2001) suggested that nearly 80% of females with lymphedema experienced its onset within three years after surgery (axillary lymph node dissection [ALND]). According to Petrek and associates (2001), females without lymphedema for three years post-surgery have almost 1% chance per year of developing lymphedema for at least 20 years. Advancements in surgical procedures (sentinel lymph node biopsy [SLNB]) is used to reduce the prevalence of lymphedema. A sentinel node is a node to which tumour cells are likely to migrate from a primary tumour. If the sentinel node is invaded, lymphadenectomy is performed with the subsequent risk of lymphedema increased. Breast cancer lymphedema typically affects the hand or arm of the same side of the body and is a result of dissection and irradiation of the axillary lymph nodes (Mortimer, (1998).
​
Unfortunately, some medical professionals advise patients to avoid intense upper body exercise due to causing lymphedema (Knols et al., (1996)). However, there is at present no available evidence to support an association between upper body exercise and breast cancer-associated lymphedema (Cheem and Gaul, 2006; MacKenzie et al., 2003; Hojan et al., 2016). In contrast, Schmitz and associates (2009) reported that compared to a control group that performs no training the incidence of upper body swelling was comparable, and the severity of self-reported lymphedema was reduced. Additionally, the incidence of lymphedema exacerbation was lower in breast cancer patients performing resistance training. Lane and colleagues (2007) noted that the reduction in lymphedema may be due to some physiological changes linked with long term physical activity and exercise. According to Mackenzie (1998) increases in sympathetic outflow and increased muscular contractions could aid in lymph return to the blood and the recruitment of dormant lymph vessels as a result of exercise.
Effects of Exercise During and After Cancer Treatment

Physical activity and exercise as a component of care for cancer patients is an acknowledged method to treat cancer-related fatigue, fitness loss and the adverse psychological effects of cancer treatment (Mishra et al., 2014; Scott et al., 2018). Exercise interventions can occur at various stages across the cancer control continuum (Courneya et al., 2007). This section concentrates on the role of exercise during four post-diagnosis stages: pre-treatment [prehabilitation], treatment, survivorship and end of life (Figure 2).
​
Pre-treatment includes the stage after a conclusive cancer diagnosis and the period until first treatment commences (which may range from weeks to several years). Silver (2015) stated that cancer prehabilitation represents “a process on the continuum of care that occurs between the time of cancer diagnosis and the beginning of acute treatment. It includes physical and psychological assessments that establish a baseline functional level, identifies impairments, and provides targeted interventions that improve a patient’s health to reduce the incidence and the severity of current and future impairments”. The treatment stage comprises the primary cancer treatments including surgery, radiation therapy, chemotherapy and biological therapies and may last months to years. Survivorship is the period following first diagnosis and initial treatment and before cancer recurrence or end of life. The end-of-life period focuses on those cancer survivors with reduced life expectancy and are receiving palliative care. Consideration should be given in the context of exercise prescription including the goals, individual’s motives, barriers, risks, and training dosages (i.e., modes, volumes, duration, progression) will vary across the cancer control continuum.

Figure 2. The cancer care continuum.
Exercise performed during and after cancer treatment is an effective tool that may improve functional capacity (VO2max), muscular strength, mobility, fatigue, psychological wellness and health-related quality of life in cancer patients and survivors (Knols et al., 2005; Gerritsen and Vincent, 2015). Knols and associates (2005) however reported that the benefits of exercise vary due to the type of cancer and treatment, stage of cancer, the modality of the exercise program, and lifestyle of the individual. Numerous studies performed on adults have concentrated on breast cancer (Table 5), prostate cancer (Table 6) and other types of cancer. However, there is inadequate research in this field and much more research is required.
Table 5. Summary of exercise studies conducted during or after breast cancer treatment.

Training Studies
​
Several studies have investigated exercise interventions in cancer patients and survivors have concentrated on aerobic training (Courneya et al., 2003; Windsor et al., 2004; Daley et al., 2007; Yang et al., 2015; Jones et al., 2014). During and after treatment aerobic intervention studies in adults have suggested a reduction in improved functional capacity or aerobic performance, improved functional mobility, improved body composition, reduced fatigue, increased psychological well-being, and improved hematologic and immune system variables (Table 5 and Table 6).
​
Several studies have investigated the effects of resistance training on cancer patients and quality of life (Segal et al., 2003; Adamsen et al., 2003). Additionally, other studies have examined the effects of combining aerobic and resistance training and others comparing both training types. Unfortunately, the is inadequate evidence available that investigates specific training variables on training outcomes. Studies that have examined resistance training and combined aerobic and resistance intervention during and after cancer treatment have displayed positive improvements in several areas (Table 5 and Table 6).
Table 6. Summary of exercise studies conducted during prostate cancer treatment.
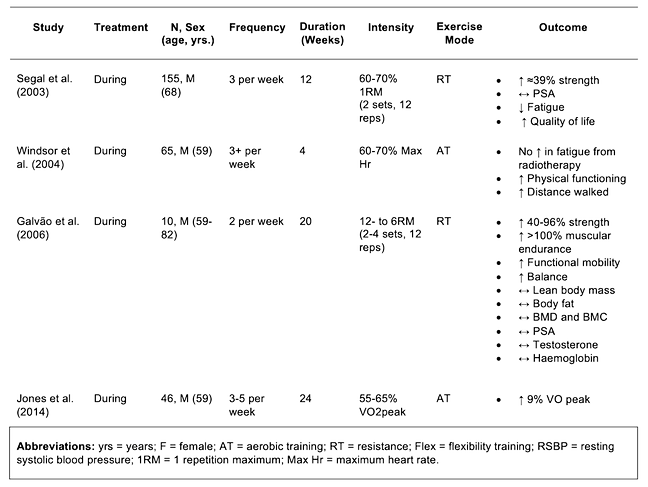
Exercise Testing & Prescription

Exercise Testing
Individuals who have been diagnosed with cancer and curative cancer treatments face significant challenges to their body systems including performing exercise and/or being affected by exercise. For example, survivors of breast cancer who have had lymph nodes removed may respond differently to inflammation and injury on the side of the body that experienced surgery. These aspects have considerable implications for exercise testing and programme design. It is important to consider that cancer and cancer therapy may conceivable affect the health-related components of physical fitness (i.e. cardiorespiratory fitness, muscular strength and endurance, body composition, and flexibility) as well as neuromotor functioning.
Understanding how an individual has been affected both physiologically and psychologically by their cancer journey is vital before the commencement of any exercise testing or exercise programme design. Every individual with cancer may have had an exclusive personal experience and subsequent response. Due to the range of experiences in this population, the safety guidelines for pre-exercise assessments of cancer survivors should be applied. There should be greater importance placed on general and cancer site-specific recommendations of the medical assessments (Table 7).
​
ACSM 2010 roundtable initially established exercise testing recommendations from empirical evidence and their own clinical and research experience. The recommendations suggested are based on the backdrop of existing guidelines (ACSM, 2009). This has since been redeveloped with Table 8 presenting recommendations for pre-exercise medical assessments and exercise testing for cancer survivors. Fitness professionals need to be conscious of the toxicities associated with certain cancer treatments in addition to the increased fractures and cardiovascular events that may ensue with hormonal therapies, neuropathies related to types of chemotherapy and also cardiotoxicity. Cancer survivors with metastatic disease to the bone will also require modifications (e.g. reduce impact loading, intensity and volume) to the exercise prescription to reduce the risk of skeletal fractures.
Cancer survivors should generally undergo a comprehensive assessment of all components of health-related physical fitness (i.e. cardiorespiratory fitness, muscle strength and endurance, body composition, and flexibility), with some specific cancer-specific considerations, to individualise an appropriate exercise prescription. However, requiring a comprehensive physical fitness assessment before commencing exercise may produce unnecessary barriers. Riebe et al. (2018) state that most cancer survivors do not require assessments to commencement a low-intensity aerobic training, resistance training with gradual progression, or a flexibility program. However, Riebe and colleagues noted that medical clearance may still be required and is conditional on exercise and health history and the presence of cardiovascular, renal, or metabolic symptoms.
​
Table 7. ACSM 2010 Pre-exercise Medical Assessment Recommendations.

Table 8. ACSM 2019 Exercise Testing Recommendations.

Exercise Prescription
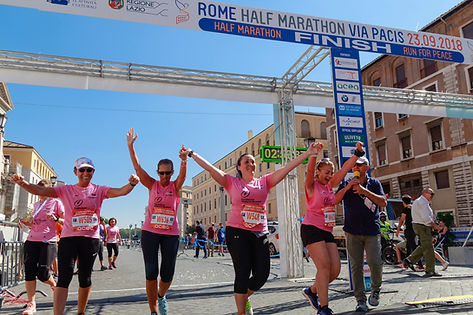
The American College of Sports Medicine in 2010 arranged a roundtable meeting of experts in the area of cancer and exercise. The central aim of these discussions was to formulate an initial set of recommendations for cancer survivors (Schmitz et al. 2010). Evidence was derived from studies that focused on breast and prostate cancer survivors, the primary outcomes from these scoping reviews were that exercise training was largely safe during and after cancer treatment. Additionally, there was evidence that exercise could yield significant health outcomes for some cancer survivors. The data gathered suggest that exercise could improve physical fitness, physical functioning, quality of life, and cancer-related fatigue. However, the evidence was from a restricted number of applicably robust studies and there was inadequate evidence to inform of specific exercise prescriptions for any health and physical outcomes nor by cancer site or treatment mode.
​
This ultimately leads to the development of guidelines that followed the 2008 Physical Activity Guidelines for adults with chronic conditions. These recommendations advised that a minimum of 150 minutes per week of aerobic activity and that resistance training be performed on two or more days a week and the performance of daily stretching of major muscle groups. The use of specific exercise testing and program modifications were founded on the patient's health status and cancer and the treatment-related side effects (Department of Human and Health Services, 2008 [Table 9]). Cancer survivors were urged to become as physically active as possible and attempt to avoid physical activity (Schmitz et al. 2010).
Table 9. DHHS (2008) Physical Activity Guidelines for Cancer Survivors.
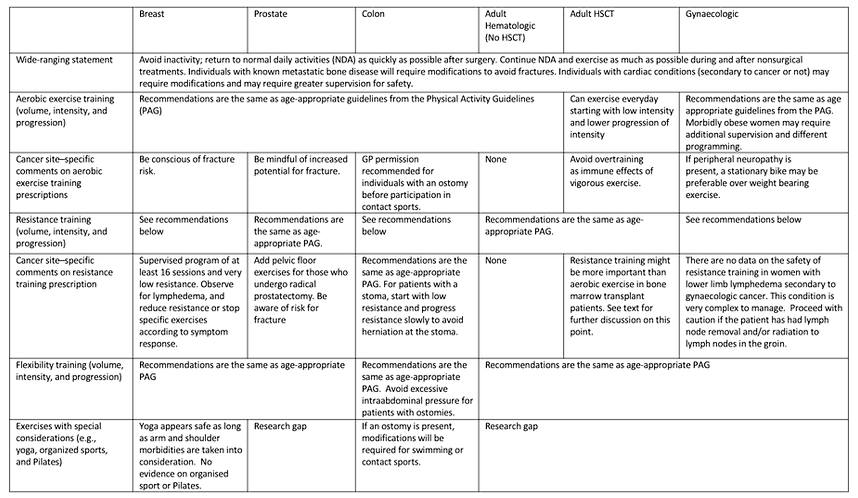
The ACSM 2010 exercise prescription guidelines (Table 10) were developed against the backdrop of other published exercise guidelines on cancer (Doyle et al. 2006; Haskell et al. 2007; Nelson et al. 2007; PAGAC, 2008). Universally these recommendations have established that exercise prescription should be individualised according to the pre-aerobic fitness, response to treatment (and negative effects) and medical comorbidities that are experienced. Table three notes the objectives for exercise, cancer site-specific contraindications and injury risk management. Additionally, goals include improving body composition (i.e. maintaining and increasing muscle mass) and decreasing body fat during and after treatment. There are considerations, however, as survivors of certain cancers (i.e. head and neck, gastric cancers, or oesophageal) may be underweight at the commencement of treatment and may further reduce weight as a result of treatment. Although early-stage prostate and breast cancer survivors are overweight obese at the time of diagnosis and may also further increase fat accumulation during treatment.
ACSM recommendations are also in agreement with the need to avoid inactivity and survivors should attempt to return to normal daily activities as soon as feasibly possible after surgery and during initial cancer treatment. Age-appropriate guidelines for aerobic activity are viewed to be applicable for survivors with some cancer site-specific considerations regarding the conceivable raised risk of skeletal fractures and infection among specific survivors who receive particular types of treatments. ACSM (2010) stated that exercise during and after treatment from the studies reviewed is safe for most types of cancer treatment, including bone marrow transplant treatments. Resistance and flexibility exercises are also recommended for cancer survivors, with modifications suggested for those with and at risk for lymphedema, and risk fractures (e.g. those with osteoporosis or bony metastases) and infection risk among those who are immune-compromised because of treatment. However, ACSM (2010) acknowledged that specific research examining the safety of strengthening and flexibility activities is limited presently. The safety and efficacy of alternate types of exercise such as yoga, Pilates, or sporting activities have not been well studied, so it is difficult to make recommendations for these activities.
Table 10. ACSM 2010 Exercise Prescription for Cancer Survivors.

ACSM 2010- Breast Cancer Key Recommendations
ACSM 2010 recommendations for breast cancer and exercise are founded on randomised control studies (RCT) that met the following inclusion criteria:
-
Concealment of those individuals assessing eligibility cannot influence treatment assignment,
-
The similarity of groups at baseline on prognostic and outcome measures
-
Standardisation of interventions with all subjects in each specified group receiving the same treatment intervention
-
>70% exercise adherence per study
-
≤ 20% attrition per study
-
Blinding outcome assessors to randomisation outcome
-
Comparable timing of outcome assessment in all groups
-
Studies on exercise during versus after chemotherapy or radiation are presented separately.
​
During Chemotherapy or Radiation Therapy
Twenty-two RCT met the inclusion criteria with reported high internal validity that have examined the safety and efficiency of exercise training among breast cancer survivors during chemotherapy or radiation treatment. However, not all RCT’s focused entirely on breast cancer with some including other cancer types. The study sample sizes ranged from 20-to-450 subjects with a mean sample of 88 women per study.
Safety of Exercise Training
From the 22 reviewed RCT studies on exercise training and the safety of breast cancer survivors during treatment, 13 reported adverse events during treatment. However, all 22 studies concluded that exercise was safe during breast cancer treatment.
Aerobic Fitness
Ten RCT studies met the ACSM pre-set inclusion criteria. Outcomes for aerobic fitness suggested that there was a strong body of evidence (Category A) that exercise training has significant improvements in cancer survivors aerobic capacity (Table 12). The pooled evidence ranged from studies that were home-based walking programs to structured and supervised exercise sessions including aerobic, resistance and flexibility components.
Table 12. ACSM 2010 cited studies for aerobic fitness and reported findings.
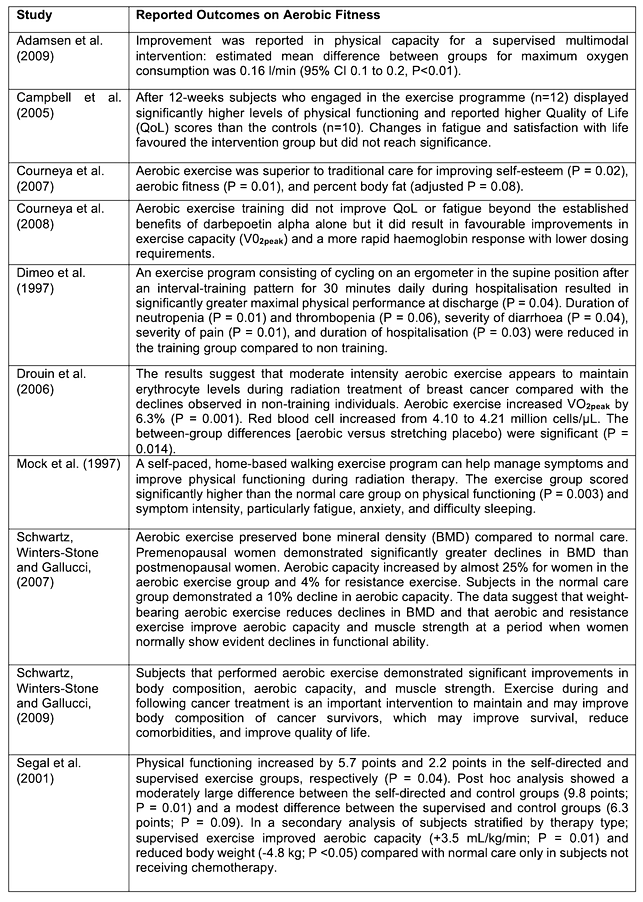
Muscular Strength
Five studies on muscular strength (Table 13) met the inclusion criteria and reported that exercise training on muscular strength during breast cancer treatment had statistically significant effects. However, it is important to note that not all these training interventions incorporated both aerobic and muscular strength training activities.
​
Table 13. ACSM 2010 cited studies for muscular strength and reported findings.

Body Size and Composition
Six RCTs were reviewed that assessed the effects of exercise on body composition during breast cancer treatment (Table 14). From the six included studies (see table), two reported no effect of exercise on body composition or body size (Battaglini et al. 2007; Demark-Wahnefried et al. 2008). However, three studies noted that percentage body fat was improved ( Battaglini et al. 2007; Courneya et al. 2007; Schwartz and Winters-Stone, 2009) with a further two studies (Battaglini et al. 2008; Demark-Wahnefried et al. 2008) reporting that body weight was reduced and increased lean mass in breast cancer survivors compared to normal patient care.
Table 14. ACSM 2010 cited studies for body size and composition and reported findings.
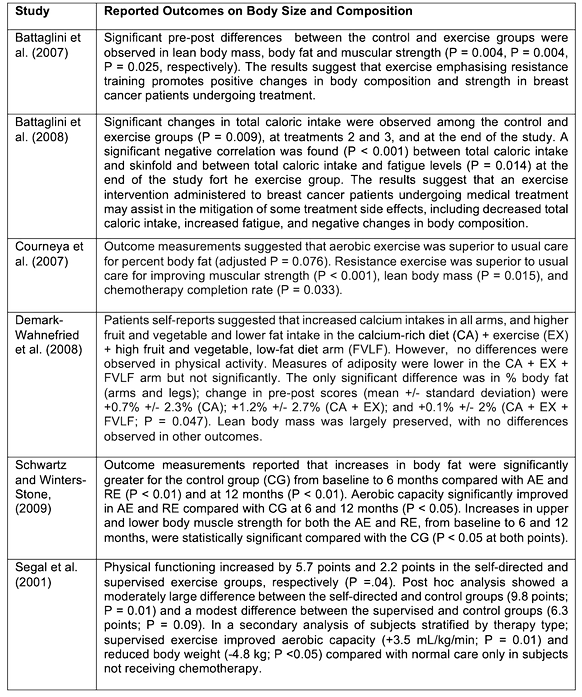
Fatigue
Seven RCT studies were cited (Table 15) that examined the efficacy of exercise to moderate fatigue during chemotherapy. The results were mixed with four studies suggesting that exercise had a significant positive effect on fatigue (Dimeo et al. 1999; Headley, Ownby and John, 2004; Mock et al. 1997; Schwartz and Winters-Stone, 2009) with three demonstrating no effect or no statically significance (Battaglini et al. 2008; Courneya et al. 2007; Mock et al. 2004). Additionally, the included study by Courneya et al. (2009) which had the largest sample size (n = 242) reported that aerobic or resistance training had no effect on fatigue during chemotherapy for breast cancer survivors.
Table 15. ACSM 2010 cited studies for fatigue and reported findings.
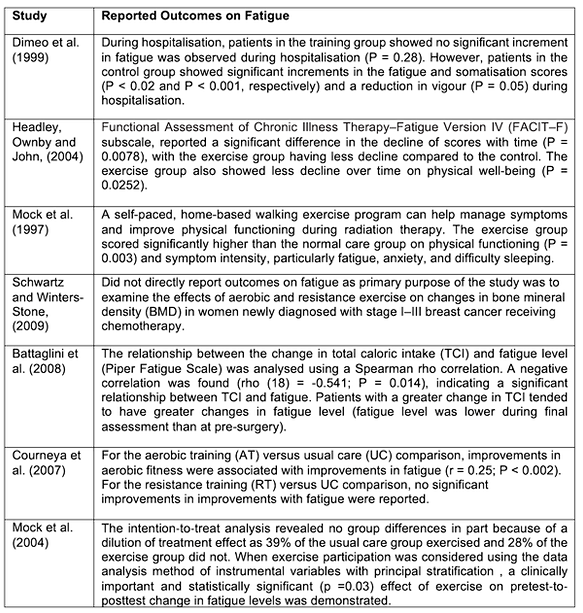
After Chemotherapy or Radiation
The ACSM (2010) cited 32 RCTs deeming them to have high internal validity that assessed the safety and efficacy of exercise training in breast cancer survivors
who have completed surgery, chemotherapy, and radiation therapy. Study sample sizes ranged from 14-to-306, with a mean of 86.
Safety of Exercise Training
From the 32 studies cited by ACSM (2010) on safety, 15 discussed the safety or adverse events. All 15 studies reported that exercise was safe for breast cancer survivors to perform after treatment. However, in one study (Kilgour, Jones and Park, (2008) 25% of subjects in the home-based intervention for shoulder rehabilitation had to discontinue after two weeks due to swelling. Practitioners should be aware that the prevalence of long term shoulder injury in breast cancer survivors is between 35-58% and care should be taken regarding specific testing and exercise prescription.
Aerobic Fitness
Twelve RCT studies met the ACSM pre-set inclusion criteria (Table 16) . Outcomes for aerobic fitness suggested that there was a strong body of evidence (Category A) that exercise training has significant improvements in cancer survivors aerobic capacity. The pooled evidence included the timed distance tests and maximal oxygen consumption to determine improvements in subjects aerobic capacity. Data from all but two studies (Bennett et al. 2007; Fillion et al. 2008) reported statistical significant improvements in aerobic capacity compared to control subjects.
Table 16. ACSM 2010 cited studies for aerobic fitness and reported findings.
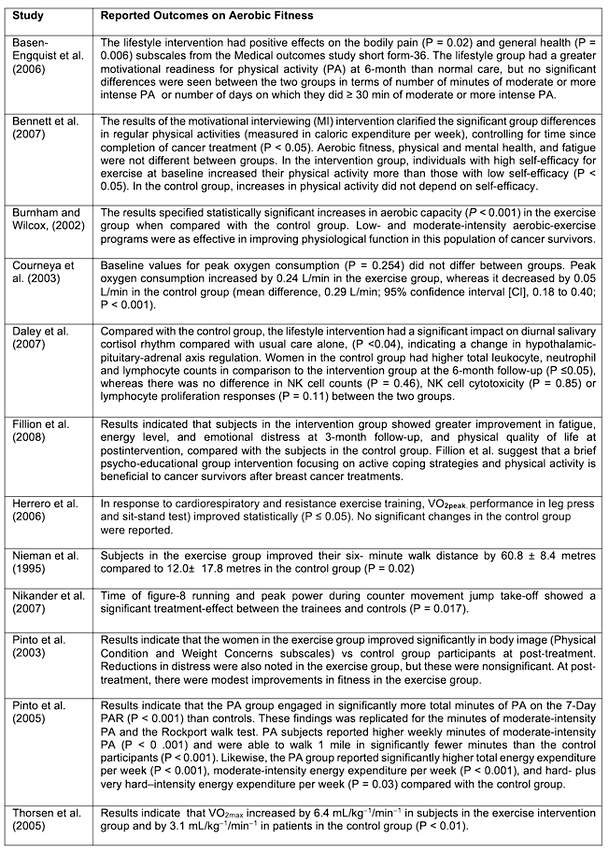