
Cardiovascular Disease: An Introduction
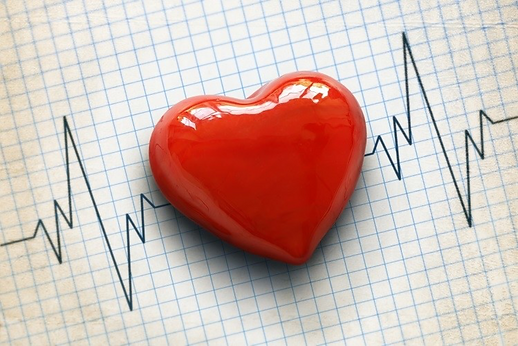
Table 1. Deaths from and numbers living with heart and circulatory diseases (CVD)
Introduction​
The term cardiovascular disease (CVD) includes several conditions that affect the healthy workings of the heart. These conditions include coronary artery disease, myocardial pumping dysfunction, electrical conduction irregularities and valvular disease (Figure 1). There are several modifiable and non-modifiable risk factors related to the development of CVD including high levels of blood-borne low-density lipoproteins and triglycerides, hypertension, smoking, obesity, type 2 diabetes, low cardiorespiratory fitness and physical inactivity (Paffenbarger et al. 2001). Physical inactivity and low levels of cardiorespiratory fitness have been reported to be independent risk factors. The main non-modifiable risk factors include genetic family history, ethnicity, gender and the individual's age. Henrisken (1999) has also identified that poor foetal health and low birth weight are strong predictors of CVD.
​
The financial burden associated with poor public health has been recognised by the United Kingdom and other highly developed industrial countries. This includes public health initiatives that seek to encourage the nation to eat healthily, avoid excess consumption, increase physical activity levels and become physically healthier (Depart of Health, 2019)

Figure 1. Cardiac disease.
Epidemiology of Cardiovascular Disease
​
The World Health Organisation’s (WHO) states that CVD is the number one cause of death globally. The global estimate of deaths by CVD (including cardiac disease, strokes and vascular diseases) stands at 17.9 million (based on 2016 data). This represents 31% of all global deaths, with 85% of these a result of a heart attack or stroke. In the United Kingdom, approximately 7.4 million people live with heart and circulatory disease. Unfortunately, this is twice as many as people living with cancer and Alzheimer’s disease combined. This accounts for 27 per cent of all deaths in the UK (167,000 deaths each year) (BHF, 2020). Additionally, around 80% of people with CVD have at least one other health condition.
​
Since 1961 premature deaths from overall cardiovascular disease (CVD) and specifically coronary (artery) heart disease (CHD) have decreased. However, the premature (under 75) death rate for Glasgow City (138 per 100,000) is approximately four times higher than that for Hart in Hampshire (39 per 100,000). Premature deaths from heart and circulatory diseases (before the age of 75) are most common in the north of England, central Scotland and the south of Wales, and lowest in the south of England (Table 1).
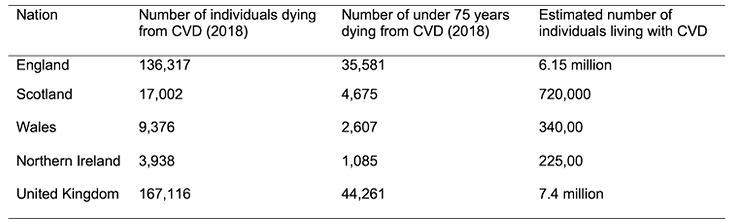
The continuing pooling of data shows that CVD in the UK and Europe remains a leading cause of mortality. In the UK approximately 40% of all deaths are attributable to CVD, of which half are attributable to CHD. Similar rates are reported in the USA as in the UK but in Western Europe, Australia and Japan the rates are up to 50% lower than in the UK and USA. In Eastern Europe, however, these are greater by three to four times compared with the UK. More men than women die from CHD (22% of men and 12% of women).
​
It is individuals with these conditions that form the groups of people eligible for exercise-based rehabilitation (Department of Health 2000). Data on the prevalence of cardiac disease morbidity has been stated to be difficult to gather (Petersen et al. 2005). Data gathered ranges from individuals that have survived myocardial infarctions, those living with angina, heart failure, valve disease and arrhythmia. It is people with these illnesses that form the groups of people eligible for exercise-based rehabilitation (Department of Health 2000). Secondary prevention, including risk factor modification and improved medical and surgical interventions, has been responsible for the large reduction in cardiac disease mortality over the 30 years (Tunstall-Pedoe et al 2000). However, up to 60% of this decline can still be attributed to risk factor modification (Unal et al 2004).
Pathophysiology of Hypertension

Unfortunately, over one billion people globally have hypertension and this number will undeniably increase. Evidence from the Framingham Heart Study suggests that 90% of individuals over 55 years of age will develop hypertension during their lifespan. Consequently, this disorder signifies a public health concern since it is a major risk factor for coronary artery disease (CVD), stroke, heart failure, renal disease, and peripheral vascular disease. Sixty-six per cent of hypertensive individuals are either unaware of their elevated blood pressure or have not been treated sufficiently to reduce cardiovascular risk. Furthermore, because elevated blood pressure is frequently asymptomatic until an acute cardiovascular event occurs, screening for hypertension is an essential aspect of preventive medicine.
​
Hypertension also poses a problem to the scientific and medical communities due to underlying intricacies. For example, in almost 90% of affected individuals, the source of the increase in blood pressure BP is undetermined, a condition often termed essential hypertension (EH). Evidence indicates that the causes of EH are varied, but a significant understanding of those reasons can be attained by examining the normal physiology or blood pressure control. High blood pressure that is attributed to a specific reason is termed secondary hypertension.
​
While secondary hypertension is less common than EH, conditions that trigger secondary hypertension are vital because many are amenable to treatment. This is because many of these conditions are now understood to cause secondary hypertension. As more evidence is presented more is therefore known about the pathophysiology of high blood pressure leading to fewer cases of hypertension.
​
Hypertension
An individual's blood pressure value can be varied and inclines to increase with age. The risk of a vascular complication increases with higher blood pressure values but the precise cut off point to outline various stages of hypertension are subjective.
The currently accepted criteria are detailed in Table 2. By this taxonomy, a diastolic pressure value consistently ≥ 90 mm Hg or a systolic pressure ≥ 140 mm Hg determines the diagnosis of hypertension. Those with prehypertension (systolic 120 to 139 mm Hg or diastolic 80 to 99 mm Hg) have an increased risk of developing hypertension over time. Although the importance has previously been on the level of diastolic pressure, more contemporary evidence suggests that systolic pressure more accurately forecasts cardiovascular complications.
Table 2. British Hypertension Society Classification of Hypertension
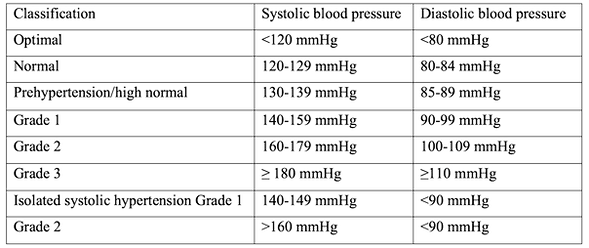
There are at least four systems that are directly responsible for the regulation of blood pressure namely the heart; the blood vessel tone; the kidneys and hormones, which regulate the functions of the other three systems. Additionally, the renal component should be discussed concerning the regulation of blood pressure as hypertension is more than just a cardiovascular problem. This is because renal excretion can return blood pressure to normal by reducing intravascular volume. Consequently, the maintenance of chronic hypertension requires renal input.
​
When an individual's kidneys function normally, an increase in blood pressure leads to elevated urine volume and also sodium excretion which returns blood pressure to normal (termed pressure natriuresis). However, hypertensive individuals have their kidneys dampened thus higher pressures are necessary to excrete water and sodium load. Existing evidence indicates several reasons for this dampened response. Firstly, tubulointerstitial and microvascular damage within the kidneys of hypertensive individuals impairs sodium excretion. Secondly, the defect may be with hormonal factors essential to appropriate renal reactions to the sodium and intravascular volume environment
Blood Pressure Reflexes
​
The cardiovascular system has several feedback mechanisms that constantly monitor arterial pressure. These receptors detect pressure changes becomes and then respond rapidly to those changes. For example, the baroreceptor reflex is one such mechanism that is mediated by receptors in the aortic arch walls and also the carotid sinuses. These baroreceptors monitor rate and changes in pressure by detecting the stretch and deformation of the arteries. If the arterial pressure increases the baroreceptors are stimulated increasing their conduction of impulses to the central nervous system. Within this loop are negative feedback signals that relay back to the circulation through the autonomic nervous system reducing blood pressure to baseline levels.
​
The more the blood pressure increases the more the baroreceptors are stretched and the greater the impulse transmission rates to the brain are. Signals from the carotid sinus receptors are transmitted by the glossopharyngeal nerve, whereas those from the aortic arch receptors are transmitted by the vagus nerve. These nerve fibres intersect at the tractus solitarius in the medulla, where the baroreceptor impulses inhibit sympathetic nervous system outflow and stimulate parasympathetic outcomes. The results are a reduction in peripheral vascular resistance (vasodilation) and cardiac output. Each of these results lowers the arterial pressure toward baseline values. Equally, when a reduction in systemic pressure is detected by the baroreceptors, fewer impulses are conducted to the medulla, leading to an automatic increase in blood pressure.
​
The outcomes of the baroreceptor mechanism are to regulate variations in systemic blood pressure. Importantly, the baroreceptor reflex is not involved in the long-term regulation of blood pressure and does not inhibit the development of chronic hypertension. This is because the baroreceptors continually reset themselves. After several days of exposure to higher than baseline pressures, the baroreceptor- ring rate reduces back to its control value leading to a new set point.

Figure 2. Blood pressure reflexes
Essential and Secondary Hypertension
​
Almost 90% of hypertensive individuals have blood pressure values that are elevated for no known reasons and are considered to have essential hypertension (EH). The identification of EH is one of elimination and is typically left to the clinician to consider the causes of secondary hypertension. Essential hypertension is typically a description than it is a diagnosis with physicians indicating only that an individual displays a specific physical outcome (i.e. high BP) or which no cause has been established. Importantly, various underlying defects are responsible for the raised blood pressure in different population groups.
​
​Although EH is more prevalent than secondary hypertension there are structural or hormonal reasons for hypertension that may be found in a small percentage of individuals. Identification of these cases of secondary hypertension is essential because the underlying conditions may require treatment that is different from EH. Furthermore, if secondary hypertension is left uninhibited, adaptive cardiovascular modifications may develop similar to those of chronic EH that could cause the increased pressures to continue even after the underlying cause is rectified.
​
Table 3. Examples of various causes of hypertension

Pathophysiology of Heart Disease: A Brief Overview
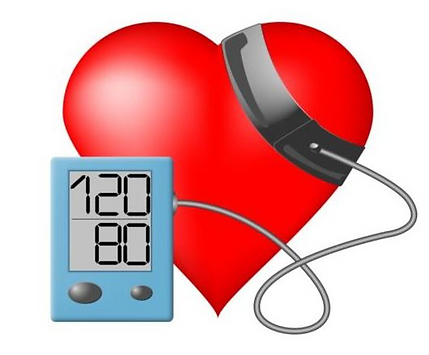
Pathophysiology of Coronary Artery Disease
​
Throughout the past decade, we have developed a greater understanding of the pathophysiology of coronary artery disease (CAD). As individuals with CAD commonly present with either chronic or acute indices, this section will discuss in turn these separate modes of presentation.
​
Lesion formation was originally considered a cholesterol storage disease. However, our present understands of atherogenesis is that of a multifaceted interaction of risk factors including the blood and cells of the artery wall and molecular communication and exchange (Libby, 2002). Evidence derived from laboratory studies has specified that inflammation plays a central role in all stages of atherogenesis. It has also been reported that inflammation contributes to the local, myocardial and systemic complications of atherosclerosis (Libby, 2002). When the arterial endothelium faces specific bacterial products or risk factors as varied as dyslipidaemia, vasoconstrictor hormones involved in hypertension, the by-products of glycoxidation related to hyperglycemia, or proinflammatory cytokines resulting from excess adipose tissue. These cells increase the expression of adhesion molecules that promote the attachment of blood leukocytes to the inner surface of the arterial wall.
​
The movement of the adherent leukocytes is dependent on the manifestation of chemoattractant cytokines controlled by signals related to conventional and developing risk factors for atherosclerosis. Once these reside in the arterial intima, the blood leukocytes (i.e. mononuclear phagocytes and T lymphocytes) correspond with endothelial and smooth muscle cells. Significant communication is transmitted among the cell types involved in atherogenesis including lipid mediators (prostanoids) and other derivatives of arachidonic acid (leukotrienes). Other autacoids, including histamine, control vascular tone and increase vascular permeability. Current research has focused on various protein mediators of inflammation and immunity (i.e. cytokines).
​
A major consequence of the inflammatory turbulence ensuing in the initial atheroma is that the smooth muscle cells migrate from the tunica media into the intima. These cells multiply and form a rich and multifaceted extracellular matrix. Together with the endothelial cells and monocytes, the smooth muscle cells secrete matrix metalloproteinases (MMPs) in reaction to several oxidative, hemodynamic, inflammatory, and autoimmune signals. Libby and Lee (2000) reported that MMPs with their endogenous tissue inhibitors, control various functions of vascular cells, including activation, proliferation, migration, and cell death. Specific elements of the extracellular matrix (namely proteoglycans) bind lipoprotein and extend their occupancy in the intima making them more vulnerable to oxidative modification and glycation (Williams and Tabas, 1998). As the lesion develops, calcification may ensue through mechanisms comparable to those in bone development. In addition to cell proliferation, cell death typically occurs in the established atherosclerotic lesion. The death of lipid-laden macrophages can lead to extracellular deposition of tissue factors. The extracellular lipid that amasses in the intima can combine and form a lipid-rich “necrotic” core of the atherosclerotic plaque.
Atherosclerosis
​
This section comprises two parts. The first part describes the ‘normal’ arterial wall, the pathogenesis of atherosclerotic plaque formation, and the complications that lead to indices of the disease. The second part explains findings from studies that have identified specific risk factors or atherosclerotic events.
Atherosclerosis is the primary cause of mortality and morbidity in industrialised countries. Through its main indices of myocardial infarction (MI) and stroke, it has also become a key cause of death in developing countries. Usually documented as “hardening of the arteries,” atherosclerosis originates its name from the Greek roots athere-, meaning “gruel,” and -skleros, meaning “hardness.” Contemporary evidence has established that chronic inflammation increases the atherosclerotic process and transduces conventional risk actors (i.e. hypercholesterolemia) into altered behaviour of vascular wall cells, contributing to the disease and its thrombotic complications. The development of atherogenesis can loiter throughout adulthood, interspersed by acute cardiovascular events.
​
Overview of a ‘Normal’ Arterial Wall
​
The arterial wall comprises of three layers (Figure 2): (1) the intima, closest to the arterial lumen; (2) the middle layer, known as the media; and (3) the outer layer, the external (also know as adventitia). A solitary layer of endothelial cells covers the intimal exterior and offers a metabolically active barrier between circulating blood and the vessel wall. The media is the thickest layer of the ‘normal’ artery. Elastin, identified as the internal and external elastic laminae, separate this middle layer from the intima and externa. The media comprises smooth muscle cells (SMCs) and extracellular matrix and aids in the elastic and contractile functions of the vessel.
​
The elastic section expands during the high pressure of systole and then recoils during diastole, pushing the blood forward. The muscular section constricts or relaxes to adjust vessel resistance and therefore luminal blood flow. The external encloses nerves, lymphatics, and blood vessels that nourish the cells of the arterial wall. Additionally, the arterial wall hosts active interchanges between its cellular components.
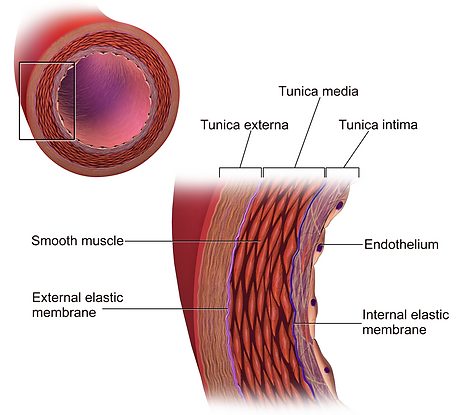
Figure 2. Diagram of the arterial wall.
Endothelial Cells
In a typical healthy artery, the endothelium performs several purposes of the vessel wall that maintain homeostasis including structural, metabolic, and signalling functions. The closely attached endothelial cells form a barrier that encloses blood within the lumen of the vessel and regulates the path of large molecules from the circulation into the subendothelial area. As blood navigates the vascular tree, it faces antithrombotic molecules produced by the endothelium that inhibit it from clotting or that promote fibrinolysis (the enzymatic breakdown of the fibrin in blood clots). Several of these molecules dwell on the endothelial surface, while other antithrombotic products of the endothelium enter the circulation. Although a net anticoagulant state typically predominates, the endothelium can also produce prothrombotic and antifibrinolytic molecules when subjected to numerous stressors. Additionally, endothelial cells secrete substances that regulate the contraction of SMC in the medial layer. These substances include vasodilators and vasoconstrictors that change the arteriolar resistance and consequently the luminal blood flow. In a ‘normal’ artery, the prevalence of vasodilator substances results in net smooth muscle relaxation.
Endothelial cells can also regulate the immune response. In the lack of pathologic stimulation, healthy arterial endothelial cells resist leukocyte adhesion and thus combat local inflammation. However, endothelial cells react to infection or local injury by expressing cell surface adhesion molecules, which link mononuclear cells to the endothelium, and chemokines. These substances enable leukocyte recruitment to the site of injury. This results in part by activation of the transcription factor nuclear factor kappa B (NFκB.)
Vascular Smooth Muscle Cells
​
Smooth Muscle Cells (SMC) have both synthetic and contractile capabilities within the medial layer of ‘normal’ muscular arteries. Several vasoactive substances regulate contractile function, resulting in vasodilation or vasoconstriction. Such agonists include angiotensin II (circulating molecules) and acetylcholine that is released from local nerve terminals, and others deriving from the covering endothelium. SMC also produce proteoglycans, elastin and collagen, which form the majority of the vascular extracellular matrix. Furthermore, SMC produces vasoactive and inflammatory mediators, including interleukin-6 (IL-6) and tumour necrosis factor (TNF). Most SMC in ‘normal’ arteries exist in the medial layer, however, human arteries also contain several in the intima, specifically in sites susceptible to atherosclerosis. Throughout atherogenesis, medial SMC can drift into the intima, proliferate, and increase the synthesis of extracellular matrix macromolecules while they diminish contractile protein content.
​
Extracellular Matrix
​
In ‘normal’ arteries, elastin, fibrillar collagen and proteoglycans compose most of the extracellular matrix in the medial layer. Interstitial collagen fibrils, composed of interconnecting helical proteins have superior biomechanical strength, with elastin providing the needed flexibility. Both these components collectively preserve the structural integrity of the vessel, despite the elevated pressure inside the lumen. The extracellular matrix also controls the development of its inhabitant cells.
​
Atherosclerotic Arterial Wall
​
The arterial wall is an active and controlled structure, however certain stimuli can interrupt homeostasis and lead the way for atherogenesis. For instance, vascular endothelial cells and SMC respond to inflammatory mediators (i.e. IL-1 and TNF) and can also produce them. With the acknowledgement that vascular wall cells react to and produce proinflammatory agents, studies into the role of “activated” endothelial and SMC in atherogenesis has increased. As a result, vascular endothelium and SMC join established inflammatory cells, including mononuclear phagocytes and T lymphocytes, as significant elements in primary atheroma formation and advanced plaque progression.
​
This essential inquiry has identified numerous components that add to atherosclerotic inflammatory development. This includes accretion of lipids within the intima, recruitment of leukocytes and SMC to the vessel wall, extracellular matrix deposition, development of foam cells and endothelial dysfunction. The cells of atherosclerotic lesions do not follow a sequential pathway but continuously interact and amend behaviour, manipulating the plaque over years into one of several conceivable profiles.
​
This segment catalogues these mechanisms into three pathologic stages: (1) the fatty streak; (2) plaque progression; and (3) plaque disruption.
Fatty Streak
​
The earliest evident signs of lesions of atherosclerosis are that of fatty streaks or deposits. They appear as regions of yellowing on the artery’s inner surface, but they do not protrude significantly into the arterial lumen nor inhibit blood flow. Interestingly, most individuals by the time they are 20 years old have fatty streaks in their coronary arteries and the aorta. However, they do not trigger any signs, and in some sites in the vasculature, they may even regress. Although the specific initiation of fatty streak development in humans is not fully understood, studies in animals have indicated that several stressors produce primary endothelial dysfunction.
​
This dysfunction permits the entry and alteration of lipids within the subendothelial space, where the lipids function as proinflammatory moderators that initiate foam cell formation and leukocyte recruitment. This is the pathological hallmark of the fatty streak.
​
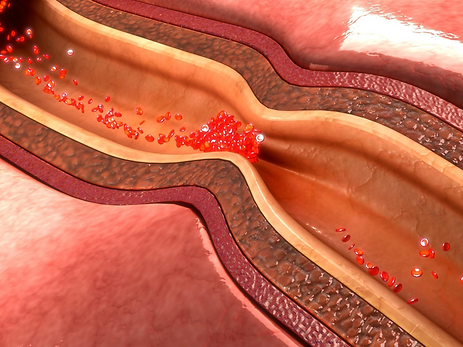
Figure 3. Atherosclerotic Arterial Wall.
Endothelial Dysfunction
​
Damage to the arterial endothelium signifies an initial occurrence in atherogenesis. Such damage can result from exposure to various agents, including physical forces and biochemical irritations. The susceptibility of key branch points of the arteries to develop atheromatous plaque supports the role of hydrodynamic trauma. In linear segments of arteries, the laminar shear forces favour the endothelial production of nitric oxide, which is an endogenous vasodilator, an inhibitor of platelet accumulation, and an anti-inflammatory substance. Furthermore, laminar movement and elevated shear stress initiate transcription factors (i.e. Krüppel-like actor 2 [KLF2]) that induces an “atheroprotective” panel of endothelial functions and heightens the expression of the antioxidant enzyme superoxide dismutase, which safeguards against reactive oxygen species. Equally, impaired flow ensues near arterial branch points, initiating low shear stress, which damages these local “atheroprotective” endothelial functions. Consequently, arteries with limited branches display relative resistance to atherosclerosis, while bifurcated vessels contain sites for atheroma formation.
​
Exposure to a noxious chemical environment may also result in endothelial dysfunction. For instance, diabetes, abnormal circulating lipid levels and smoking tobacco are all known risk factors for atherosclerosis and can promote endothelial dysfunction. These risk factors increase reactive oxygen species production specifically superoxide anion that interacts with other intracellular molecules to affect the metabolic and synthetic functions of the endothelium. As a result of this environment, the cells stimulate local inflammation. When both chemical and physical stressors disrupt normal endothelial homeostasis, an activated state develops. This is manifested by damage of the endothelium function as a barrier, resulting in the release of inflammatory cytokines, increased production of cell surface adhesion molecules that recruit leukocytes, the altered release of vasoactive bodies and interference with antithrombotic properties. These undesirable effects of dysfunctional endothelium lay the foundation for consequent events in the advancement of atherosclerosis.
Lipoprotein Access and Alterations
​
The stimulated endothelium is no longer an active barrier to the path of migrating lipoproteins into the arterial wall. The increased endothelial permeability allows greater access for low-density lipoproteins (LDL) into the intima, a process assisted by raised circulating LDL concentration in individuals with hypercholesterolemia. Furthermore, high LDL levels from the individual's diet (in part) lead to numerous monogenic causes of elevated LDL including mutations of the LDL receptor of apolipoprotein B and a protease (PCSK9) involved in the control of the LDL receptor. When LDLs are within the intima they amass in the subendothelial space by attaching to proteoglycans in the extracellular matrix. This LDL entrapment increases the time within the vessel wall, where the lipoprotein may undergo chemical alterations that can advance the development of atherosclerotic lesions. Hypertension can further increase retention of lipoproteins in the intima by increasing the production of LDL-binding proteoglycans by the smooth muscle cells.
​
One type of modification that develops with the trapped LDL in the subendothelial space is oxidation. This may be due to the local actions of reactive oxygen species and prooxidant enzymes or from macrophages that infiltrate the vessel wall. Furthermore, the micro milieu of the subendothelial space seizes oxidised LDL from antioxidants in the plasma. These organic modifications of LDL contribute to the inflammatory mechanisms introduced by endothelial dysfunction, and may also continue to aid inflammation during the lifecycle of the plaque. In the fatty streak and during plaque development, modified LDL (mLDL) promotes leukocyte recruitment and foam cell formation.
Leukocyte Recruitment
​
The recruitment of leukocytes to the vessel wall is a significant stage in atherogenesis. The progression depends on the expression of leukocyte adhesion molecules (LAMs) on the non-adherent endothelial luminal surface and on chemoattractant signals that direct diapedesis into the subintimal space. There are two important subgroups of LAM that stay in the inflamed atherosclerotic plaque: (1) the immunoglobulin gene superfamily (specifically vascular cell adhesion molecule-1 [VCAM-1] and intercellular adhesion molecule-1 [ICAM-1]); and (2) the selectins (E- and P-selectin). These LAMs and chemoattractant signals direct monocytes to the developing lesion.
​
It has been reported based on rodent models that hypercholesterolemia favours the accretion in the blood of a subgroup of monocytes that is categorised by expression of high levels of proinflammatory cytokines (IL-1 and TNF). T lymphocytes also localise within plaques and direct the adaptive immune response. Proinflammatory cytokines and mLDL can induce LAM and chemoattractant cytokine expression independently, but mLDL may also stimulate endothelial and SMC to produce proinflammatory cytokines, thus reinforcing the direct action. This dual ability of mLDL to aid in leukocyte recruitment and inflammation, directly and indirectly, continues during atherogenesis.
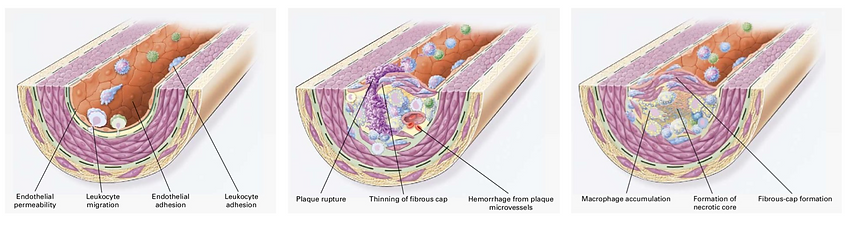
Figure 4. (Left) Endothelial dysfunction in atherosclerosis; (Middle) Fatty streak formation in atherosclerosis; (R) Formation of an advanced atherosclerosis lesion
Foam Cell Formation
​
Once monocytes adhere and infiltrate the intima, they separate into imbibe lipoproteins and macrophages to form foam cells. Macrophages rely on scavenger receptors that bind and affect mLDL. Unlike the uptake through the LDL receptor, mLDL absorption by scavenger receptors avoids adverse feedback inhibition and allows engorgement of the macrophages with cholesterol and cholesteryl ester, resulting in the manifestation of foam cells. While this uptake may be initially favourable by isolating potentially damaging mLDL elements, the compromised efflux of these cells compared to the rate of influx and local propagation, leads to their accretion in the plaque. This diminishes their protective role by stimulating foam cell apoptosis and releasing proinflammatory cytokines that encourage atherosclerotic plaque development. Throughout atherogenesis, the removal of dead foam cells is typically ineffective, consequently aiding in the build-up of cellular fragments and extracellular lipids, establishing the necrotic core (lipid-rich centre of a plaque).
​
Plaque Development
​
Unlike endothelial cells, smooth muscle cells located in the intima aid in plaque advancement by producing an extracellular matrix that entraps lipoproteins and adds to the mass of the lesion. Years of atherosclerotic plaque development leads to a distinctive thrombogenic lipid core that produces a protective cap. It is important to note that not all fatty streaks develop into fibro fatty lesions. Initial plague development involves external remodelling of the arterial wall that maintains the lumen diameter and allows plaque build-up without blood flow restriction. Lesions at this early stage, therefore, elude angiography exposure. Advanced plaque development, however, can exceed the compensatory arterial development, limit the vessel lumen, and inhibit perfusion.
​
Such blood flow restrictive plaques can result in tissue ischemia, triggering suggestions of angina pectoris or intermittent claudication of the extremities. Various acute coronary syndromes ensue when the fibrous cap of an atherosclerotic plaque ruptures, revealing prothrombotic particles inside the lipid core and causing an acute thrombus that rapidly obstructs the arterial lumen.
​
Smooth Muscle Cell Migration
​
The change from fatty streak to fibrous atheromatous plaque involves the movement of SMC from the arterial media into the intima and the subsequent increase of the SMC within the intima and excretion of extracellular matrix macromolecules by the SMC. Foam cells activated platelets entering via micro-fissures in the plaque exterior and endothelial cells can initiate elements that signal SMC resettlement and propagation.
​
Foam cells generate numerous factors that add to SMC recruitment. For instance, they release platelet-derived growth factor (PDGF) which may stimulate the passage of SMC across the interior elastic lamina and into the subintimal space where they replicate. PDGF also incites the growth of local SMC in the intima. Foam cells also release cytokines and growth factors (TNF, IL-1, fibroblast growth factor, and transforming growth actor-β [TGF-β]) that stimulate SMC and the synthesis of extracellular matrix proteins. Moreover, these cytokines stimulate SMC and leukocyte activation, increasing additional cytokine release, consequently augmenting and sustaining inflammation in the lesion.
​
Adhering to the established model, plaques develop progressively. However, contemporary evidence has indicated that this development may be interrupted by subclinical events with surges of smooth muscle reproduction. For instance, evidence from intraplaque haemorrhages specifies that fissures in plaque integrity can ensue without clinical symptoms. These plaque disturbances reveal tissue factors from foam cells to blood, which triggers clotting and micro thrombus development. Stimulated platelets within this microthrombi release additional potent factors that can stimulate a localised surge of SMC movement and proliferation. Stimulated T cells also aid in plaque development with cells of the T helper 1 subtype (Th1) producing proinflammatory cytokines. This is while lymphocytes of the T helper 2 subtype (Th2) and regulatory T cells (Treg) produce TGF-β and IL-10 which can prevent SMC proliferation and diminish plaque growth.
​
Plaque Disturbance
The mêlée between matrix synthesis and degradation endures over years but not without consequences. Death of SMC and foam cells, due to either excessive inflammatory stimulus or by the initiation of apoptosis pathways, releasing cellular substances that add to imbibed lipids and cellular fragments to the growing lipid core.
​
The magnitude of the lipid core has mechanical effects on the integrity of the plaque. With the increasing size and bulge into the arterial lumen, biomechanical stress centres on the plaque border adjoining normal tissue. The structure of the fibrous cap adds to the integrity of the plaque. Whereas lesions with thick fibrous caps may cause noticeable arterial narrowing they have less susceptibility to rupture. Equally, plaques that have thinner caps tend to be more fragile and more prone to rupture inciting thrombosis.

Figure 5. Normal artery wall vs. atherosclerotic plaque
Rupture of atherosclerotic plaque does not necessarily trigger major clinical events such as stroke or myocardial infarction. Minor non-occlusive thrombi may integrate into the plaque, promoting further smooth muscle growth and extracellular matrix accumulation. The balance between the thrombogenic and fibrinolytic potential of the plaque and the fluid phase of blood determines whether disruption of the fibrous cap leads to a transient, nonobstructive mural thrombus or to a completely occlusive clot. The prospect of a significant thrombotic episode resonates with the balance between the opposing processes of clot formation and dissolution by fibrinolysis. An individual’s susceptibility toward clotting may be heightened by genetics, comorbid conditions and lifestyle actors.
Chronic Ischemic Heart Disease
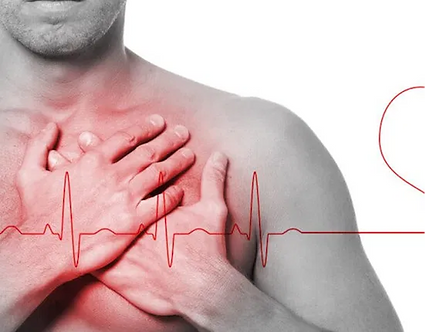
Introduction
​
Heberden in 1772, first reported a disorder in which individuals developed a tight sensation in the chest when walking. He termed this discomfort as angina pectoris and observed that this disorder would fade soon after the individual stood still but would reappear when the comparable activity started again. At the time Heberden did not fully know the cause of this disorder by it was this initial description of symptoms of ischemic heart disease. This observation refers to an imbalance between myocardial oxygen supply and demand, most often instigated by atherosclerosis of the coronary arteries. Angina pectoris is a common manifestation of ischemic heart disease and has been termed “strangling in the chest”. Other conditions may lead to similar discomfort but angina pectoris refers specifically to the uncomfortable sensation in the chest and adjacent structures that develops from the disparity between myocardial oxygen supply and demand.
​
Ischemic heart disease now afflicts millions of individuals globally and is the primary cause of death in developed nations.The clinical appearance of ischemic heart disease can be inconstant and forms a variety of conditions. For instance, ischemia may be accompanied by similar exertional symptoms depicted by Heberden. In other occurrences, it may ensue without any clinical signs, a condition termed silent ischemia. This section describes the causes and description of chronic ischemic heart disease
​
Pathophysiology of Ischemia
​
The conventional belief of myocardial ischemia in coronary artery disease is that it is a result of atherosclerotic plaques that narrow the vessel’s lumen and restrict myocardial blood supply. However, contemporary investigations have suggested that the reduction of blood flow in this condition results from the combination of fixed vessel constriction and abnormal vascular tone, contributed to by atherosclerosis-induced endothelial cell dysfunction.
​
The coronary arteries comprise large, proximal epicardial segments and smaller, distal resistance vessels (arterioles). The proximal vessels are subject to evident atherosclerosis that results in stenotic plaques. The distal vessels are typically free of flow restricting plaques and can regulate their vasomotor tone in response to metabolic demands (Figure 6). These resistance vessels operate as a reserve, increasing their diameter with exertion to meet increasing oxygen requirements and dilating, even at rest, if proximal stenosis is severe.
​
The hemodynamic consequence of a coronary artery restriction depends on both the degree of stenosis of the epicardial portion of the vessel and the amount of compensatory vasodilatation the distal resistance vessels can attain. For example, if stenosis narrows the lumen diameter by <60%, the maximal possible blood flow through the artery is not significantly changed and in response to exertion, the resistance vessels can dilate to provide sufficient blood flow. However, if stenosis reduces the diameter by >70%, resting blood flow is normal, but maximal blood flow is reduced even with full dilatation of the resistance vessels. In this situation as oxygen demand increases the coronary flow reserve is insufficient with oxygen demand surpassing supply and results in myocardial ischemia. If the stenosis reduces the vessel lumen by > 90%, then even with maximal dilatation of the resistance vessels, blood flow may be insufficient to meet basal metabolic requirements and ischemia can ensue at rest.
​
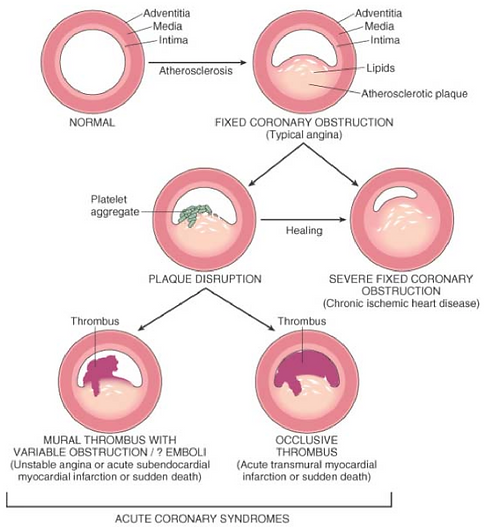
Figure 6. Schematic of coronary artery lesion progression.
Endothelial Cell Dysfunction
​
The other key contributor to restricted myocardial oxygen supply in chronic coronary artery disease is endothelial dysfunction. Atypical endothelial cell function can add to the pathophysiology of ischemia in two ways: (1) through the loss of normal antithrombotic properties; and (2) by inappropriate vasoconstriction of coronary arteries.
​
In addition to their vasodilatory activities, factors released from endothelial cells (including NO and prostacyclin) also use antithrombotic properties by delaying platelet accumulation. However, in states of endothelial cell dysfunction, the release of these substances is reduced; therefore, the antithrombotic effect is diminished. Consequently, in syndromes characterised by thrombosis the reduced release of NO and prostacyclin allows platelets to amass and secrete their detrimental procoagulants and vasoconstrictors.
In a normal individual, physical or psychological stress results in considerable coronary artery vasodilatation. This effect is controlled by activation of the sympathetic nervous system, with increased blood flow and shear stress initiating the release of endothelial-derived vasodilators (i.e. NO). It has been suggested that in normal individuals, the relaxation effect of NO offsets the direct α-adrenergic constrictor effect of catecholamines on arterial smooth muscle, resulting in vasodilatation. However, individuals with atherosclerosis and reduced release of endothelial vasodilators leave the direct catecholamine effect unimpeded, resulting in vasoconstriction. The subsequent decrease in coronary blood flow contributes to ischemia.
​
Individual’s with CAD risk factors including hypercholesterolemia, diabetes mellitus, hypertension, tobacco smoking and impaired endothelial-dependent vasodilatation have suggestive symptoms before the development of atherosclerotic lesions. These observations indicate that endothelial dysfunction occurs in the initial stages of the atherosclerotic process. Incorrect vasoconstriction seems to be an important factor in acute coronary syndromes (i.e. unstable angina and myocardial infarction). The reported reason for acute coronary syndromes is the disruption of atherosclerotic plaque, with overlaid platelet accumulation and thrombus development. Generally, the products of platelet accumulation in an evolving clot result in vasodilatation since they stimulate the endothelial release of NO. However, with dysfunctional endothelium, the direct vasoconstricting activities of platelet products prevail, further impairing flow through the arterial lumen.
​
Additional Causes of Myocardial Ischemia
​
There are other reported conditions other than atherosclerotic coronary artery disease that may lead to an imbalance between myocardial oxygen supply and demand that result in ischemia. These conditions include hypotension and decreased blood oxygen content. For instance, a person with considerable bleeding from the gastrointestinal tract may develop myocardial ischemia and angina pectoris due to the reduced oxygen supply. Conversely, on the contrasting side of the equilibrium, an extreme increase in myocardial oxygen demand can initiate ischemia even in the absence of coronary atherosclerosis. For example, this can ensue with rapid tachycardias, acute hypertension, or severe aortic stenosis.
​
The implication of ischemia exposes the insufficient myocardial oxygenation and local accretion of metabolic waste products. For instance, during ischemia, myocytes convert from aerobic to anaerobic metabolic pathways. The diminished production of ATP impairs the interaction of the contractile proteins and leads to a temporary reduction of ventricular systolic contraction and diastolic relaxation due to the energy-dependent processes. The resulting increase of left ventricular diastolic pressure is directed to the pulmonary capillaries and can increase pulmonary obstruction leading to signs of dyspnoea. Furthermore, metabolic compounds including lactate, serotonin, and adenosine accrue locally. It is thought that these compounds initiate peripheral pain receptors (C7 -to-T4) and may be the mechanism for this discomfort of angina. The accumulation of local metabolites and transient abnormalities of myocyte ion transport may also accelerate arrhythmias.
​
The consequence of ischemic events on the myocardium depends ultimately on the severity and extent of the imbalance between oxygen supply and demand. It was once assumed that ischemic cardiac damage resulted in irreversible myocardial necrosis or conversely a rapid and full recovery of myocyte function. Contemporary evidence has further added to these outcomes including ‘stunned myocardium’ and ‘hibernating myocardium’.
​
The term ‘stunned myocardium’ refers to the tissue damage caused after an event with prolonged systolic dysfunction even after the return of normal myocardial blood flow. In this situation, the functional, biochemical, and structural irregularities following ischemia are reversible and contractile function progressively improves. The mechanism accountable for this delayed recovery of function involves myocyte calcium overload and the accumulation of oxygen-derived free radicals during ischemia. Generally, the degree of stunning is relative to the degree of the prior ischemia and this is possibly the response to an ischemic event that is just short of producing irreparable necrosis.
The implication of ischemia exposes the insufficient myocardial oxygenation and local accretion of metabolic waste products. For instance, during ischemia, myocytes convert from aerobic to anaerobic metabolic pathways. The diminished production of ATP impairs the interaction of the contractile proteins and leads to a temporary reduction of ventricular systolic contraction and diastolic relaxation due to the energy-dependent processes. The resulting increase of left ventricular diastolic pressure is directed to the pulmonary capillaries and can increase pulmonary obstruction leading to signs of dyspnoea. Furthermore, metabolic compounds including lactate, serotonin, and adenosine accrue locally. It is thought that these compounds initiate peripheral pain receptors (C7 -to-T4) and maybe the mechanism for this discomfort of angina. The accumulation of local metabolites and transient abnormalities of myocyte ion transport may also accelerate arrhythmias.Conversely, the term ‘hibernating myocardium’ denotes the tissue changes due to the chronic ventricular contractile dysfunction and the subsequent reduction in blood supply, commonly due to multivessel coronary heart disease. In this state, permanent damage has not transpired and ventricular function can quickly improve if applicable blood flow is restored by surgical or percutaneous revascularization.
​
Physical Activity and Coronary Heart Disease

The causal association between physical activity levels (PAL) and chronic morbidity has been documented since the 1950s (Paffenbarger et al. 2001). Furthermore, evidence from primary and secondary prevention suggests that there is a decline of 8-17% in premature mortality from CHD for every one metabolic increase in aerobic fitness (Franklin and Gordon, 2005, Myers et al. 2004). For clarification one MET is the amount of oxygen used each minute per kilogram of body mass while sitting at rest. The mean aerobic capacity of a 50-year-old European adult is 8-10 METS and this declines by almost 2 METS per decade (Astrand et al. 2003, Hollenberg et al. 2006). McPherson et al. (2002) has reported that physical inactivity increases a greater risk of CHD than hypertension, smoking and obesity. It was only high cholesterol values that were reported to be a more potent risk factor. Furthermore, reduced or impaired physical fitness is as a strong predictor of CVD as an individuals family history of CHD, hypertension, high cholesterol, obesity and smoking (Farrell et al. 1998, Laughlin, 2004).