Developing Muscular Power
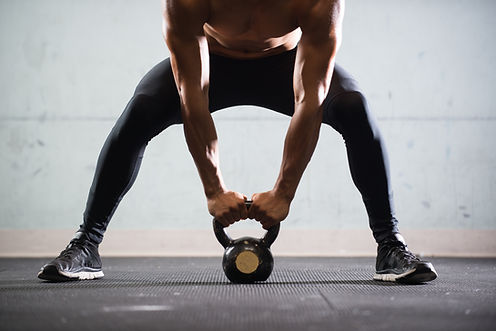
Introduction
Understanding the nature of power is central to sports performance. Investigating power from its beginning at the molecular level offers important information when designing athletic training programs. Force production is often viewed as being one-dimensional in its translation to performance. However, power is a multidimensional system of force, displacement, velocity, and work. Examining power at a molecular level gives valuable insight into ways of developing effective methods that maximize the athletic performance of the whole-body system. This is specifically true when we consider the external environment in which we have evolved since the inception at the molecular level provides valuable information when designing an optimal training program. Force production is considered one-dimensional in its translation. However, power appears to be a multifaceted system of force, displacement, velocity, and work. These variables should also be put in the context of the constantly changing system in which we examine this phenomenon in terms of muscle lengths, joint angles, and concentric, eccentric, and stretch-shortening cycle patterns of muscle function. Examining power at a molecular level provides a valuable context in which to develop effective ways to maximize the athletic performance of the body as a whole, specifically within the environment in which we have evolved (exclusive of body mass, gravity, and air resistance).
​
Defining Power
​
Power has been defined as the rate of performing work and is a product of force and displacement. Another way to think about power is the amount of force produced during an activity at a given velocity. As discussed in the S & C online lecture the myosin–actin interaction (cross-bridge) has been referred to as the power stroke. Power is the culmination of force, displacement, and time. These three variables are central to what defines athletic performance, and thus, why it has been widely studied and discussed by both sports scientists and practitioners.
​
Movement patterns including ballistic or semi-ballistic actions (i.e. jump squat and the power clean) have been suggested to yield higher power outputs in contrast to heavy-weight (i.e. higher % of 1RM) squats (Cormie et al., 2007; Garhammer, 1993; McBride, Haines & Kirby, 2011). Although heavy bench presses and squats appear to involve comparatively high force, the velocity of the movement appears to be lower than the explosive press-up, jump squat, or power clean. This lower velocity has been suggested to result in lower power values (McBride, Haines & Kirby, 2011). Physical activities performed at very high velocities also appear to produce lower power outputs due to the force being low according to the force-velocity relationship in the muscle.
Nevertheless, a condition of very high velocity that limits power is not a naturally occurring condition in human movement, except if completed in zero-gravity or microgravity environments. It has been suggested that running and jumping within the earth’s environment produces relatively high-power outputs because we move our whole body against the gravitational force of the earth (i.e. our own body weight) (Cavagna et al., 1972; Methenitis et al., 2016). This suggests that a condition of moderate levels of velocity and force may occur concurrently, as commonly reflected in data from the squat jump using body mass. This is an interesting notion as the early work from Cavagna et al., 1972 initially reported this when subjects performed jumping in simulated zero-gravity or microgravity environments. If you perform a maximal squat, power output is generally low (high force, low velocity). If you jump or run on the earth, your power appears to be high (moderate force, moderate velocity). However, if you jump on the moon, your power is low (low force, high velocity). This hyperbolic relationship may aid in establishing ideas of why and where power ensues in human movement and how to train in order to maximize athletic performance.
​
Understanding the Importance of Power
​
Power is vital for running quickly and jumping high. If athletes want to jump higher or run faster, they must produce maximal force via maximal displacement in a short period. In addition, they must do this by moving their own body mass against gravity. It is important to consider that our body mass is a force that must be overcome to generate power. Therefore, a cross-bridge, a muscle fibre, a whole muscle, a joint movement, and the ground reaction force may be improved in the context of this environmental setting. Interestingly, the concept of maximal force, velocity, and power production may be observed from the level of an individual muscle fibre to a whole muscle, to a joint, and lastly to the whole body (Miller et al., 2015; Plas et al., 2015).
Power output capability is an outcome of the maximal force a system can yield. As discussed in the S & C online lectures previously, hydrolysis of adenosine triphosphate (ATP) has been reported to result in free energy, and therefore, mechanical work, all within a specified period. However, the production of force, whether it be in a single cross-bridge or a whole muscle, must be placed in the environmental setting of what the maximal force production capability of the system is (i.e. velocity). This is because power is a product of force and velocity, thus, the optimum juncture between these two variables may provide a better understanding of where power ensues and how it can be enhanced (Plas et al., 2015).
​
Understanding Maximal Power
​
A study by Finley and colleagues (2015) examined a single muscle fibre and suggested that maximal power may occur at 15-to-30% of its maximal force capabilities, with others suggesting that it may translate to the whole muscle. Furthermore, it could be proposed that this may translate into the force output of the whole body. For example, if an athlete weighs 85.8 kg (841 Newtons [N]) and they can produce 167.9 kg (1647 N) of maximal force with the legs in a vertical direction, then the external load at which the athlete can create the most power vertically (jump squat) appears to be 33.8% [of this total value ([841 N + 1647 N] × 33.8% = 840.9 N).
The answer to the equation is 840.9 N, which is almost the weight of the athlete and 33.8% of the total value (2,488 N), which is similar to the values of 15-to-30% of maximal force production reported in studies of single muscle fibres (Fitts & Widrick, 1991; Nuzzo et al., 2010). This suggests that athletes can generate the most power when they are moving a load approximately equal to their own body weight. McBride, Haines, and Kirby (2011) reported that when the training load increases, peak force increases with decreases in peak velocity and peak power.
Therefore, ballistic power training may be characterized by training with lower resistances (high peak power) and strength training may be characterized by training with higher resistances (high peak force) (Soriano et al., 2015). This association may be somewhat different when using the squat or power clean as a modality for power training (Soriano et al., 2015), in that the loading is heavier and expressed as a percentage of how much weight the athlete lifts (bar weight or one repetition maximum [1RM]). In the case of the squat, it might be 56% of 1RM, and in the power clean it might be 80% of 1RM (Soriano et al., 2015). Every so often, the terms of load are different between the jump squat, squat, or the power clean. For example, the loading for the jump squat is the athlete’s body weight (BW) as the load or the athlete’s body plus some level of additional external loading (i.e. weighted plates). The loading for the squat or the power clean traditionally has been placed with how much load is on the bar (1RM).
​
Assessment of Athlete's Power
Within the strength and conditioning literature power is one of the most debated aspects of human performance. In the strength and academic literature power has been assessed through an array of modes (e.g. isokinetic, isoinertial, and ballistic), using various loads, and during an assortment of exercises. These assessments and measurements are usually performed to describe human muscular performance. However, the traditional methods of measuring power output have often occurred during repeated maximal efforts over a distance or for a given amount of time as with a stair-climbing test or Wingate power test (Findley, Chaudhry, and Dhar, 2015). Recently, measuring power output has been performed using during ballistic exercises. These are frequently defined as throwing and jumping actions that allow the athlete to accelerate the body or a bar through the complete range of motion (Croce et al., 2014). Ballistic exercises are not power activities entirely but are physical activities in which external mechanical power or system power is of a higher value than in other activities that occur at lower velocities.
​
The variable that is most measured during these ballistic exercises is the net power of the system which has been shown to be a depiction of the summed co-ordinated effort of the individual joint powers (Finni et al., 2003). Such measurements have applied benefits (e.g. cost, time, and equipment) over the direct assessment of individual joint powers. It is essential that we note that power is a measurement and the equivalent of a typical measurement of athletic performance (e.g. sprint time or vertical jump height). For example, the use of power as a measure of performance in jumping is misguided (Cormie et al., 2007) due to the variance between system power and performance. In this case, jump height is not large in either male or female athletes (Desmedt and Godaux, 1977). However, two athletes with the same jump height (performance) but different magnitudes of power offer additional information about the process of jump performance.