
Introduction
Using the Quality and Outcomes Framework and Scottish Diabetes Survey (now decommissioned) statistics with estimates from the Diabetes Prevalence Model 2016 (Public Health England) and 2012 APHO Diabetes Prevalence Model, there are a reported 4.8 million people with diabetes in the UK. This accounts for over 3.9 million people diagnosed with diabetes mellitus (Table 1) and a further 1 million are estimated to have type 2 diabetes but have not been diagnosed (Diabetes UK, 2019).
Table 1. Diabetes Prevalence in the UK (Diabetes UK, 2019)
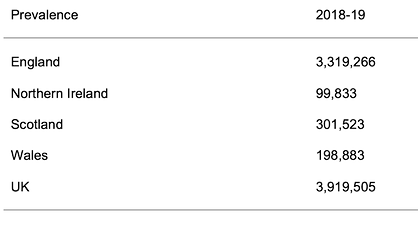
Type 2 Diabetes

Type 2 diabetes refers to a disease of insulin resistance. When individuals typically have a mixed meal the blood glucose become elevated and the pancreatic beta cells secrete insulin an important hormone that aids in the uptake of glucose into muscle, fat and other cells. Without sufficient insulin secretion and adequate blood insulin levels or with compromised insulin action (or both), blood glucose increases to abnormal levels.
Obesity is frequently associated with type 2 diabetes due to the influence of the adipokines and their pro-inflammatory response, in addition to the direct inhibitory effect of obesity on insulin receptors in muscle cells (Lenz and Fornoni, 2008). As insulin resistance advances the pancreatic insulin-producing cells create less insulin, subsequently leading to insulin deficiency and type 2 diabetes. Insulin resistance combined with other metabolic dysfunction (Despres et al., 2008) also triggers dyslipidaemia in many individuals with type 2 diabetes. It has been suggested that insulin resistance is initially present before the development of type 2 diabetes. Insulin resistance progression to the diagnosis of type 2 diabetes can take up to 10-years. Unfortunately, the clinical diagnosis of type 2 diabetes can be delayed after the initial insulin resistance development with the pathophysiology and related complications negatively affecting tissues during this period (Buysschaert et al., 2015; Kurihara et al., 2015). Eberhardt and colleagues (2004) observed that over 75% of type 2 diabetes occurrences among obese and inactive clients. Furthermore, Colditz et al., (1995) reported that individuals with a BMI of >35 have almost a 30-fold greater risk of developing type 2 diabetes compared with a BMI of <23.
The definitions and classification of normal, impaired, and diabetes levels of fasting and random or postprandial blood glucose are displayed in Table 2.
Table 2. American Diabetes Association Classification of Normal, Impaired, Diabetic Fasting and Random Blood Glucose

Epidemiology and Pathophysiology of Type 2 Diabetes: An Overview
Approximately 90% of people with diabetes in the UK have type 2, 8% have type 1 and almost 2% have rarer types. Diabetes mellitus is a significant global burden for health care. There are nearly 463 million people globally and, in the UK, approximately 5.6% of the adult population, aged between 20-to-79 have the condition (International Diabetes Federation (IDF) Atlas 2019). Throughout the UK there are over 3.8 million individuals with a diagnosis of diabetes mellitus and a projected one million people who have the condition but are unaware of it (Diabetes UK, 2019 Facts and Stats). This represents almost 8.5% of the UK population (Public Health England [PHE], 2020).
There are four common categories of diabetes:
-
Type 1, which is insulin-dependent and considered to be the result of an autoimmune attack on the beta cells of the pancreas leading to their destruction.
-
Type 2, which is predominantly the result of insulin resistance.
-
Gestational diabetes, which arises during pregnancy.
-
Diabetes due to other specific causes including monogenic diabetes (i.e., neonatal diabetes mellitus and maturity-onset diabetes of the young), drug-induced diabetes, and exocrine pancreatic deficiency (which may lead to cystic fibrosis-related diabetes).
Hill and colleagues (2013) have reported that type 2 diabetes is directly and positively associated with the mean increase in body weight over the past 25 years decades. Kaufman (2006) has used the term “diabesity” for type 2 diabetes due to the strong positive correlation with obesity. It has been reported that high levels of glucose are connected with numerous secondary complications including the following:
-
Coronary artery disease, congestive heart failure. Marwick et al., (2009) stated that 70% of individuals with diabetes die of heart disease.
-
Cerebral vascular incident (stroke).
-
Hypertension: Centres for Disease Control and Prevention (2014) stated that 71% of adults >18 years with diagnosed diabetes have a blood pressure value of ≥ 140/90 mmHg or are on antihypertensive medications.
-
Kidney disease: Centres for Disease Control and Prevention (2014) stated that diabetes was recorded as the primary cause of end-stage renal disease in 44% of newly reported cases in 2011.
-
Elevated triglycerides and low HDLs are related to insulin action on adipose cells to increase storage and insulin resistance (Feingold et al., 2015).
-
Eye disease: Diabetes is a significant cause of retinopathy and blindness (Centres for Disease Control and Prevention, 2014).
-
Peripheral artery disease: Centres for Disease Control and Prevention (2014) reported that 60% of nontraumatic amputations occur in individuals with diabetes-related to reduced blood flow and reduced healing.
Effects of Exercise in Clients with Type 2 Diabetes
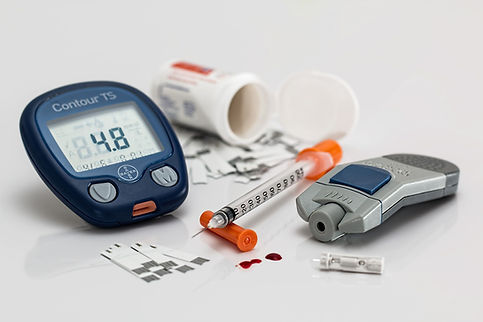
Physical activity and exercise have been indispensable components of the prevention and management of type 2 diabetes. Several studies (Colberg et al., 2016; Sigal et al., 2004) have demonstrated that increased physical activity and engagement in exercise improves insulin-dependent muscle glucose uptake independently of weight loss or a hypoglycemic diet. A study by O’Gorman et al., (2006) examined the impact of acute and short-term exercise training on whole-body insulin-mediated glucose disposal and signal transduction along the canonical insulin signalling cascade. The authors reported that short-term exercise training increased insulin-mediated glucose disposal in obese type 2 diabetic (p<0.05), but not in obese non-diabetic subjects. Glucose transport protein 4 (GLUT4 protein) was increased in obese type 2 diabetic subjects (p<0.05), but not in obese non-diabetic subjects following chronic exercise. A systematic review performed by Wang et al., (2009) examined morphological and metabolic adaptations within the skeletal muscle to exercise training in adults with type 2 diabetes mellitus or impaired glucose tolerance. A total of 18 studies met the inclusion criteria. Results suggested positive adaptations to exercise in muscle fibre area and capillary density, glycogen, glycogen synthase and GLUT4 protein expressions. However, the authors reported the need for more robustly designed exercise.
Effects of Exercise and Recommendations for Clients with Type 2 Diabetes
The benefits of regular exercise are significantly enhanced when combined with weight reduction (5-7%) and a low-fat, high-fibre diet (Knowler et al., 2002). In the Diabetes Prevention Program Outcomes Study (2009), the lifestyle intervention was significantly better than metformin in reducing the risk of developing type 2 diabetes. In adults older than 55 years, lifestyle alterations resulted in a 71% decrease in the risk of developing type 2 diabetes.
Studies have reported that resistance exercise can be a safe and when combined with aerobic exercise and can be more effective as aerobic training alone in the prevention and management of type 2 diabetes. For example, Davidson et al., (2009) examined the independent and pooled effects of resistance and aerobic exercise on insulin resistance and functional limitations in 136 abdominally obese older subjects aged 60-80 years. Subjects were randomised to 1 of 4 groups for 6 months: resistance exercise, aerobic exercise, resistance and aerobic exercise (combined exercise), or non-exercise control. Adjusting for age, sex, and baseline value, insulin resistance improved compared with controls in the aerobic exercise and the combined exercise groups but not in the resistance exercise group. Functional limitation significantly improved in all groups compared to the control group. Improvement in the combined exercise group was greater than in the aerobic exercise group but not in the resistance exercise group. Improvement in the resistance exercise group was not different from the aerobic exercise group. The authors concluded that a combination of resistance and aerobic exercise was the best exercise approach for concurrent reduction in insulin resistance and functional limitation in previously inactive, abdominally obese older adults.
This is further supported by Grøntved et al., (2014), who examined the association of muscle strengthening activities with the risk of type 2 diabetes in females. The authors performed a 8-year longitudinal study and followed up 99,316 middle-aged and older women from the Nurses’ Health Study ([NHS] aged 53-to-81 years, 2000-to-2008) and Nurses’ Health Study II ([NHSII] aged 36-to-55 years, 2001-to-2009). The subjects were free of diabetes, cancer, and cardiovascular diseases at baseline. Subjects reported weekly time spent on resistance exercise, lower intensity muscular conditioning exercises and aerobic moderate and vigorous physical activity at baseline and in 2004-to-2005. During 705,869 person years of follow-up, 3,491 incident type 2 diabetic cases were established. Resistance exercise and lower intensity muscular conditioning exercises were each independently associated with lower risk of type 2 diabetes in pooled analyses. Females who performed at least 150 min/week of aerobic moderate and vigorous physical activity and a minimum of 60 min/week of muscle-strengthening activities had substantial risk reduction (RR) compared with inactive women (pooled RR=0.33 [95% CI 0.29–0.38])
Long-term studies of at least six months have reported that resistance training may increase fat-free mass, resulting in increased insulin receptors and capacity for glucose uptake. Resistance training has also been suggested to stimulate glucose uptake and lower glycosylated haemoglobin in a similar way to aerobic exercise. Current evidence has revealed the superior benefits on insulin resistance and glycaemic control of combined resistance training and aerobic exercise in individuals with type 2 diabetes. For instance, Bacchi et al., (2012) examined the differences between the effects of aerobic and resistance training on haemoglobin (HbA1c) levels and other metabolic risk factors in subjects with type 2 diabetes. Forty type 2 diabetic subjects were randomised to either an aerobic or resistance training group. Before and after four months of exercise intervention, metabolic phenotypes, body composition, visceral and subcutaneous adipose tissue, cardiorespiratory fitness, and muscular strength were measured. After the exercise intervention, increases in peak oxygen consumption (VO2peak) was greater in the aerobic group (time-by-group interaction P = 0.045), whereas increase in strength was greater in the resistance group (time-by-group interaction P < 0.0001). HbA1c was reduced similarly in both intervention groups. The total and truncal fat, visceral and subcutaneous adipose tissue were also comparably reduced in both groups, whereas insulin sensitivity and lean limb mass were increased. β-Cell function showed no significant changes. Bacchi and colleagues concluded that resistance training is equivalent to aerobic training in improving metabolic features and insulin sensitivity and decreases abdominal fat in type 2 diabetic subjects.
Additionally, Church et al., (2010) reported the positive benefits of combining aerobic and resistance training on HbA1c in individuals with type 2 diabetes. This RCT involved 262 inactive adult subjects with type 2 diabetes and HbA1c levels of 6.5% or higher who enrolled in the 9-month exercise program. Forty-one subjects were assigned to the non-exercise control group, 73 to resistance training 3 days a week, 72 to aerobic exercise in which they expended 12 kcal/kg per week; and 76 to combined aerobic and resistance training in which they expended 10 kcal/kg per week and engaged in resistance training twice a week. The main outcomes were that compared with the control group, the absolute mean change in HbA1c in the combination training exercise group statistically significant (P= 0.03). The mean changes in HbA1c were not statistically significant in either the resistance training (P= 0.32) or the aerobic (P= 0.14) groups compared with the control group. Only the combination exercise group improved maximum oxygen consumption (P < 0.05) compared with the control group. All exercise groups reduced waist circumference (from −1.9 to −2.8 cm) compared with the control group. The resistance training group and combination training group significantly lost fat mass compared with the control group (P< 0.05).
Presented in Table 3 is the NSCA recommendations for exercise and clients with type 2 diabetes. Importantly, exercise professionals need to be conscious of updated screening guidelines, contraindications and precautions in individuals with type 2 diabetes. All clients with type 2 diabetes should be appropriately advised before an individualised exercise program.
Table 3. NSCA Recommendations for Clients with Type 2 Diabetes

Precautions, Contraindications and Exercise Modifications for Clients with Type 2 Diabetes
There are numerous recommendations and guidelines (ACSM, 2014; Colberg et al., 2010; Marwick et al., 2009) that the exercise professional should consider and be aware of when working with clients who have type 2 diabetes including the following:
-
Clients with type 2 diabetes need to be cleared medically before beginning a vigorous exercise program and should undertake a comprehensive medical evaluation, especially if they have cardiovascular, kidney, nervous, renal, or visual complications or have ≥10% risk of a cardiac event over 10 years (ACSM, 2014; Colberg et al., 2010).
-
Clients with type 2 diabetes who have > 10% risk of a cardiac event over the next 10 years should undertake a medically supervised maximal clinical exercise test before beginning an exercise program (ACSM, 2014; Colberg et al., 2010).
-
Clients with type 2 diabetes are susceptible to silent ischemia and again may need to have a clinical exercise test that detects areas of the heart with ischemia (Marwick et al., 2009).
-
Hypoglycaemia is a common but abnormal response to exercise. This has been clinically defined as a blood glucose of <70 mg/dl and specifically in clients with type 2 diabetes on insulin or multiple oral hypoglycaemic agents (Colberg et al., 2010; Marwick et al., 2009).
Clients should not engage in exercise when exogenous insulin action is peaking. Therefore, the exercise professional must be acquainted with hypoglycaemic oral medication and insulin preparations that a client will go through including:
-
Blood glucose monitoring should be performed before and after an exercise session and when starting or progressing an exercise program (Colberg et al., 2010).
-
If pre-exercise blood glucose is<100mg/dl, it is prudent to have the client ingest 20 to 30 g of carbohydrate before starting exercise (recheck glucose 10 minutes after ingestion).
-
Avoid injecting insulin into exercising limbs; it is best to use an abdominal site if a type 2 diabetes client presents with blood glucose above 200 mg/dl. It is also practical to check ketone bodies in urine. If no ketone bodies are present, light to moderate exercise is possible (30% to <60% VO2 or heart rate reserve), but vigorous exercise (≥60-to-90% VO2 or heart rate reserve) should be avoided.
-
Be mindful of dehydration, especially if the client is hyperglycaemic.
-
For clients with retinopathy, avoid vigorous-intensity exercise and excessive elevations in blood pressure (Colberg et al., 2010). Clients with autonomic neuropathy may have a dampened blood pressure and heart rate response to exercise. These clients should be referred to a medically supervised program.
-
Clients with type 2 diabetes who have kidney disease, peripheral artery disease, or peripheral neuropathy also should be referred to a medically supervised program.
-
Exercise professionals should be cautious with vigorous exercise in all clients with type 2 diabetes, as a high percentage have undiagnosed atherosclerosis of the coronary and peripheral arteries.
Type 1 Diabetes

Type 1 diabetes (also known as juvenile or insulin-dependent diabetes) is a chronic autoimmune disease that causes the body to destroy pancreatic beta cells that produce and store insulin. As a consequence, exogenous insulin is required to compensate for the limited amount of endogenous insulin being produced.
Type 1 Diabetes Epidemiology and Pathophysiology: An Overview
Type 1 diabetes (also known as juvenile or insulin-dependent diabetes) is a chronic autoimmune disease that causes the body to destroy pancreatic beta cells that produce and store insulin. As a result, exogenous insulin is required to compensate for the limited amount of endogenous insulin being produced. The highest incidence of type 1 diabetes occurs during puberty with the typical onset established by 30 years of age. However, it should be noted that type 1 diabetes can occur at any age. The American Diabetes Association (2014) has acknowledged that the exact cause of this autoimmune disease has not been fully identified. That said there are several factors related to greater prevalence including heredity and environmental influences.
Individuals with type 1 diabetes require exogenous insulin by injections or an infusion pump to regulate their blood glucose levels. Limited insulin results in the individual developing hyperglycaemia if not treated. High blood sugar levels increase the risk of dehydration due to increased urine production and if left unregulated can lead to ketoacidosis. When the body fails to take in the glucose from the blood into the cells it uses protein and fat for metabolism with ketones being produced as a by-product of fat. This accumulation of ketones reduces the blood pH levels leading to ketoacidosis producing the following common symptoms:
-
Excessive thirst or dry mouth
-
Increased urination
-
Vomiting and nausea
-
Abdominal pain
-
Shortness of breath or rapid breathing
-
Acetone (fruity-smelling) breath
-
Muscle weakness
-
Mental confusion
Individuals are frequently unaware that they have type 1 diabetes until they seek advice from a physician or other medical health professional for symptoms linked to ketoacidosis. This leads to tests including a fasting blood glucose test or an HbA1c test (Mayo Clinic, 2016). An HbA1c test shows the patient's previous two- or three-month average blood glucose level by assessing the per cent of glucose attached to blood haemoglobin. The American Diabetes Association (2012) has classified an HbA1c level of ≥6.5% assessed on two separate occasions, signifies a diabetic condition. However, Stöppler (2016) states that it does not distinguish between type 1 and type 2 diabetes. Additionally, if someone is pregnant, an HbA1c test does not provide accurate results.
Effects of Exercise and Recommendations For Clients with Type 1 Diabetes
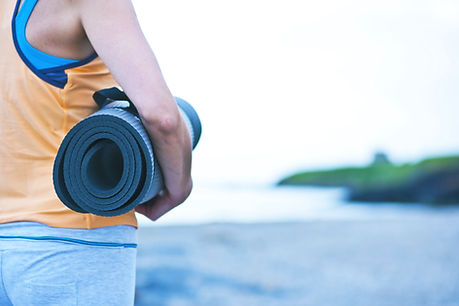
Several studies have cited the positive effects of exercise on individuals with type 1 diabetes including reduced HbA1c levels (Sideravičiūtė et al., 2006), improved body composition (Laaksonen et al., 2002; Norris, Carroll and Cochrane, 1990; Ramalho et al., 2006), reduced high blood pressure and elevated blood lipid levels (Laaksonen et al., 2000; Norris, Carroll and Cochrane, 1990), and decreased mortality rates (Moy et al., 1993).
For example, Sideravičiūtė et al., (2006) examined the effects of a 14-week swimming program on 14–19-year-old girls with and without type 1 diabetes. Nineteen subjects participated in this study with weight, height, and four skinfold thickness were measured, body mass index (kg/m2) and body fat mass (%) were computed, blood lipid levels (total cholesterol, high-density/low-density lipoprotein, and triglyceride) were estimated. Aerobic capacity was assessed by the Ruffie test. Results of the 14-week program showed that both groups improved their aerobic capacity (p<0.01 in healthy and p<0.05 in diabetics, respectively). No changes were observed in body mass index, but body fat mass decreased significantly in both healthy (p<0.001) and diabetics (p<0.001) subjects. No significant changes in blood lipid profile were found in all subjects, only high-density lipoprotein concentration increased significantly in healthy girls (p<0.001).
Despite the benefits, studies have reported that exercise can cause hypoglycaemia during exercise (Guelfi et al., 2005; Heyman et al., 2007) or after the session or hours later during sleep (nocturnal hypoglycaemia). Guelfi and colleagues (2005) compared the response of blood glucose levels to intermittent high-intensity exercise (IHE) and moderate-intensity exercise (MOD) in subjects with type 1 diabetes. Seven healthy subjects with type 1 diabetes were measured on two different occasions, during which either a 30-min MOD or IHE protocol was performed. MOD comprised of continuous exercise at 40% Vo2peak, while the IHE protocol involved a mixture of continuous exercise at 40% Vo2peak combined with 4-s sprints performed every 2 min to replicate the activity patterns of team sports. Results showed that both exercise interventions resulted in a reduction in blood glucose levels. However, this decline was greater with MOD (-4.4 +/- 1.2 mmol/l) compared with IHE (-2.9 +/- 0.8 mmol/l; P < 0.05), despite the subjects engaging in a greater volume of total work with IHE (P < 0.05). During 60 min of recovery from exercise, glucose levels remained higher in IHE compared with MOD (P < 0.05). Moreover, glucose levels remained constant during recovery from IHE, while they continued to decrease after MOD (P < 0.05). The stabilisation of blood glucose levels with IHE was related to elevated levels of lactate, catecholamines, and growth hormone during early recovery from exercise (P < 0.05). There were no significant differences in free insulin, glucagon, cortisol, or free fatty acids between MOD and IHE.
This is supported by Heyman et al., (2007) who examined the responses in sympathoadrenergic activity during intense exercise in 13-to-18 girls with type 1 diabetes. The authors found that during exercise, plasma glucose stayed constant although plasma insulin decreased in the healthy girls, whereas glucose diminished significantly with no change in plasma insulin in type 1 diabetic subjects. Throughout the exercise protocols, catecholamines increased comparably in both groups. Nevertheless, at rest and during all stages of exercise, norepinephrine levels were significantly lower while epinephrine levels were significantly higher in type 1 diabetic compared to healthy subjects.

Studies have also demonstrated that exercise can cause hypoglycaemia after the session or several hours later during sleep (nocturnal hypoglycaemia) (MacDonald, 1987; Tsalikian et al., 2005; Maran et al., 2010). Maran et al., (2010) examined the glucose profiles in non-trained subjects during and 20-hours after a session of two different modes of exercise. Eight males’ subjects with well-controlled type one diabetes volunteered for the study. Each subject underwent 30 minutes of intermittent high-intensity exercise (IHE) and moderate-intensity exercise (MOD) in random order. Expired air was documented during exercise, while metabolic and hormonal were performed before and 120 minutes after exercises. Continuous glucose and activity monitor were applied for the subsequent 20h. The authors reported that subjects blood glucose levels declined during both types of exercise. Subjects plasma glucose content was marginally greater after IHE while no differences were observed in plasma insulin concentration at 150 minutes following the start of exercise. It was noted that during IHE there was a significant increase in norepinephrine concentrations. Blood glucose levels were reported to be significantly lower after IHE than MOD between midnight and 6:00 a.m. The number of hypoglycaemic incidents after IHE was higher than that observed after MOD.
It should be pointed out that not all exercise produces a hypoglycaemic response. Studies by Tansey et al., (2006) and Tsalikian et al., (2005) have reported that only moderate-intensity exercise resulted in lower blood glucose levels during or after exercise while high-intensity interval training (HIIT) caused a postexercise increase in blood glucose or at least less of a decrease (Bally et al., 2016; Dubé, Lavoie, and Weisnagel, 2013; Iscoe and Riddell, 2011; Tonoli et al., 2012). Additionally, HIIT has been shown to lower the incidence of nocturnal hypoglycaemia (Iscoe and Riddell; Taplin et al., 2010) but not in all studies (Maran et al., 2010).
Iscoe and Riddell (2011) compared the physiological responses and related glycaemic changes to continuous moderate-intensity exercise vs. continuous moderate-intensity exercise + intermittent high-intensity exercise in athletes with Type 1 diabetes. Interstitial glucose levels were measured in a blinded approach during two sedentary days and during two days in which 45 minutes of afternoon continuous moderate-intensity exercise occurred with or without intermittent high-intensity exercise. The total amount of work performed, and the duration of exercise was equal between sessions. During exercise, marked differences between trials were observed in heart rate, respiratory exchange ratio, oxygen utilisation, ventilation and blood lactate levels were higher during continuous moderate-intensity + intermittent high-intensity exercise vs. continuous moderate-intensity exercise (all P < 0.05). Despite these differences between trials, there was no significant difference in the reduction of interstitial glucose or plasma glucose levels between trials. Nocturnal glucose levels were greater in continuous moderate-intensity + intermittent high-intensity exercise and in sedentary vs. continuous moderate-intensity exercise (P < 0.05). Compared with continuous moderate-intensity exercise alone, continuous moderate-intensity + intermittent high-intensity exercise was associated with less post-exercise hypoglycaemia and more post-exercise hyperglycaemia. Iscoe and Riddell stated that continuous moderate-intensity + intermittent high-intensity exercise protect against nocturnal hypoglycaemia in athletes with Type 1 diabetes.
Notably, most of the studies that examined the effects of exercise on type 1 diabetes were founded on subjects performing aerobic exercise. The effects of resistance training on blood glucose levels are less certain (Tonoli et al., 2012), but research by Yardley et al., (2013) has indicated that it can contribute to reducing the incidence of exercise-induced hypoglycaemia. Yardley et al., (2013) examined the acute effects of resistance exercise on glycemia during exercise and in the following 24-hours compared with aerobic exercise and no exercise. Twelve active subjects with type 1 diabetes completed 45 min of resistance exercise (3-sets of 7 exercises at 8 RM), 45 min of aerobic exercise (running at 60% of V02max), or no exercise on different days. Plasma glucose was measured during and 60 min after exercise. Interstitial glucose was measured by continuous glucose monitoring (24 h before, during, and 24 h after exercise). Significant changes were reported in decreased plasma glucose levels (P = 0.008) during resistance exercise and aerobic exercise (P = 0.001). However, no significant changes were observed during the no-exercise control session. During recovery, glucose levels did not significantly change after resistance exercise but did increase after aerobic exercise (P = 0.023). Mean interstitial glucose from 4.5 to 6.0 h postexercise was significantly lower after resistance exercise vs. aerobic exercise. The authors concluded that during resistance exercise the initial decline in blood glucose is less compared to aerobic exercise. However, resistance exercise is associated with more sustained reductions in postexercise glycemia than aerobic exercise.
Exercise Recommendations for Clients With Type 1 Diabetes
The American Diabetes Association (2016) recommends that a client with type 1 diabetes should aim to achieve 150 minutes a week of moderate- to vigorous-intensity aerobic exercise accomplished via three or more weekly sessions, two or three non-consecutive days a week of resistance training, and two or three days a week of flexibility and balance training (American Diabetes Association (2016). See Table 4 for typical exercise guidelines that are specific to type 1 diabetes.
Table 4. American Diabetes Association (2016) Exercise Recommendations for Clients with Type 1 Diabetes

Precautions, Contraindications and Exercise Modifications for Clients with Type 1 Diabetes
One of the fundamental aims regarding exercise and type 1 diabetes is to effectively balance the quantity and timing of insulin administration with pre-exercise nutrition and the mode, duration and intensity of the exercise session. Hypoglycaemia whether during or post-exercise can be controlled or avoided by the following: (1) increasing the time between the pre-exercise insulin administration and the beginning of the exercise session; (2) reducing the quantity of pre-exercise insulin (3) increasing the quantity of carbohydrate consumed before exercising; (4) lowering the amount of post-exercise insulin; or (5) a combination of these. (Chitty et al., 2008; Garg et al., 2006; Tansey et al., 2006).
The choice regarding how much carbohydrates to eat (if any) before exercise is built on the client’s pre-exercise blood glucose levels and the constraints of the approaching exercise session:
-
If pre-exercise glucose levels are < 100mg/ dl, consume 15-to-30 g of carbohydrate unless the duration of the exercise session will be < 30 minutes or at a very high intensity (no extra carbohydrate is needed) or if the session will be long (consume 0.5-1 g of carbohydrate per kilogram of body weight per hour) (American Diabetes Association, 2016).
-
Pre-exercise glucose levels between 90-to-150 mg/dl frequently require carbohydrate to be ingested from the beginning of the session and throughout the session at a rate of 0.5-to-1 g of carbohydrate per kilogram of body weight per hour (American Diabetes Association, 2016).
-
If pre-exercise glucose levels are between 90-to-150 mg/dl, delay consuming carbohydrates until levels fall under 150 mg/dl (American Diabetes Association, 2016).
-
Pre-exercise glucose levels between 250-350 mg/dl require a ketone test. If there are moderate-to-high ketone levels, the exercise session should not be performed. If the ketones are low, then light-to-moderate intensity exercise is acceptable. However, glucose levels are required to be under 250 mg/dl before performing high-intensity exercise (American Diabetes Association, 2016).
-
If pre-exercise glucose levels are >350 mg/ dl, assess ketones and delay the exercise session if they are moderate to high. If ketones are negative (or at trace levels), appropriate insulin correction as detailed by the client’s health care professional is necessary. If ketones are in an acceptable range, mild- to moderate-intensity exercise can commence, but the intensity should not be high until glucose levels are <250 mg/dl (American Diabetes Association, 2016).