Plyometric Training For Sport: An Introduction

Defining The Term Plyometrics
​
Plyometric training is a popular method used to improve athletes' performance. The rationale for this inclusion into the athletes' training plan is that it acts as a link between strength and sport-related power. Plyometric training involves a stretch of the muscle-tendon unit (MTU) followed immediately by a shortening of the muscle unit. The process of muscle lengthening and rapid shortening is termed the stretch-shortening cycle (SSC) and is integral to plyometric training. It has been reported that the SSC process significantly enhances the athletes' MTU to generate maximal force in the shortest period of time (Rassier and Herzog, 2005; Bobbert et al., 1996)
​
Plyometric training has evolved from the original model of shock training with agreement still to be reached on the terms and descriptive terminology to explain this method. Intriguingly, the translation of the Greek word pliometric (plio = more, plythein = increase, metric = measure), means “to increase the measurement.” Fred Wilt, an American track and field coach from the University of Iowa is often credited with coining the term plyometric in the modern era. ​Early academic literature described plyometric training by other names including the stretch-shortening cycle. Sports coaches in countries such as Italy, Sweden, and Russia, sports coaches referred to plyometrics as jump training. Plyometric training was initially developed as a shock method of training where Verkhoshansky believed that athletes needed to be presented with a stimulus that was unique and different from their normal training methods.
​
There is still some confusion about the terminologies used with both plyometric and stretch-shortening cycles often used synonymously to describe physical actions. In the physiology literature, the term stretch-shortening cycle is used to describe physical actions including running, throwing, and jumping. However, in the strength and conditioning literature, plyometric is the preferred term used to describe these physical activities when they are part of the training cycle designed to manipulate the SSC for maximizing force production.
To add to the further misunderstanding of terms is amortization which describes the plyometric activity. Radcliffe and Farentions (1985) described amortization as a “gradual extinction, extinguishing, or deadening”. If we use depth jump to explain amortization it can be defined as the time from the athlete's initial ground contact to take off and as a transition between eccentric (lengthening) and concentric actions (shortening) of antagonistic muscle groups.
​
We will term plyometric exercises as activities that involve and exploit the mechanisms of the SSC to increase the efficiency of force production at a joint or to increase athletic performance. In basic terms, plyometric exercises enable a muscle to reach maximum strength in as short a time as possible (also known as power).
Plyometric Exercise Physiology
​
There is a strong body of physiological research that supports the effectiveness of plyometric training. Most research indicates that there are two important factors. The first factor to consider is the serial elastic components of the muscle which include the cross bridging characteristics of actin and myosin and the tendons. Secondly is the proprioceptors in the muscle spindles that are central for pre-setting muscle tension and transmitting sensory input related to quick muscle stretching for activation of the stretch reflex.
​
When we endeavour to recognise how the stretch-shortening cycle (SSC) mechanism aids in power production one must consider the role of muscle elasticity. The skeletal muscles can temporarily store the tension that is developed by rapid stretching and possess potential elastic energy. For example, if we consider a muscle like an elastic band and whenever stretch it is stretched it has the potential for a rapid return to its original length. Another mechanism that is integral to the SSC is the stretch reflex (also known as the myotactic reflex). An example of this reflex is the patellar knee jerk (Figure 1) response when the quadricep tendons are tapped by a physician’s rubber mallet. This impact to the knee area causes the quadriceps tendon to stretch as a result this stretch is detected by the quadricep muscles which produces a muscle contraction in response.

Figure 1: Patellar Knee Reflex
The stretch reflex responds to the rate at which the muscle is stretched and is one of the quickest reflexes in the body. For this reason, the sensory receptors are directly coupled [in the muscles] to cells in the spinal cord and replay information to the muscle fibres responsible for muscular responses. There are other reflexes that are slower than the stretch reflex due to the speed and transmission through various interneuron channels and the central nervous system (CNS) before a reaction is stimulated. Due to the minimal time delay in the stretch reflex the muscle experiences action faster during an SSC than in any other mode of action. This is contrary to a voluntary response to a muscle stretch as it would be too late to effectively use for jumping or throwing activities.
​
Furthermore, consideration must be made regarding the strength of these stretch responses when determining how plyometrics can relay to athletic performance. Although the stretch reflex response time remains the constant even after training, training will alter the strength of the response in terms of the muscle response or action. When a muscle is stretched there is the potential for greater concentric force after the stretch which can be improved with increases in the rate of stretching the muscle. This resultant contraction of a rapidly stretched muscle is a more powerful movement for overcoming the inertia of an object (i.e. overcoming body weight).
The Various Stages of the Plyometric Exercise
The description of various stages of the plyometric exercise has been another area that creates confusion in the strength and conditioning literature. There have been various descriptions used to describe the various phases including biphasic (consisting of phases for eccentric and concentric muscle action) or triphasic (with an additional phase for the transition between the eccentric and concentric phases). Siff (2004) however, has further separated plyometric exercise into five phases by adding a momentum phase at the beginning and end of the triphasic description. For a more detailed explanation see Chmielewski et al., 2004

Figure 2: Phases of the plyometric exercise.
To help with consistency we will use a triphasic description of the movement. The terminology for the phases may be eccentric, amortization and concentric actions (Figure 2).
​
The Plyometric Loading Phase
​
The preliminary phase of any plyometric movement, which includes rapid muscle lengthening, is termed the loading phase. This phase has also been termed as the eccentric, deceleration, countermovement, or cocking phase. This terminology varies depending on the plyometric movement the coaches or authors are describing.
In basic terms the loading phase of a plyometric exercise occurs when the muscle-tendon units (MTU) of the agonists and synergistic muscle groups are lengthened (stretched) as a result of loading or kinetic energy applied to the joint. The kinetic energy may be derived from the concentric action (countermovement) of the antagonistic muscle group.
​
The stretching of the MTU during the loading phase elicits the SSC and results in enhanced force production and performance when compared to the absence of a stretch. The initial loading phase begins when the MTU starts to resist gravity or the prior movement. This is often termed negative work, especially in the resistance training literature. This phase is completed when the point at which the centre mass reaches the lowest position (i.e. during a jumping movement) and the velocity of the centre of mass reduces to zero.
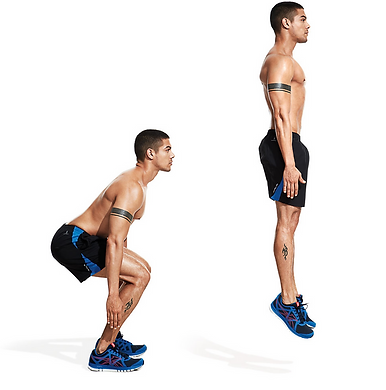
The stretch of the active muscle or muscles through the loading phase can produce increased force output via three related mechanisms associated with the stretch-shortening cycle; ([1] muscle potentiation; [2] the stretch reflex and; [3] the series elastic components). Each of these mechanisms will be discussed below.
​
Muscle Potentiation
Muscle potentiation is a change of the muscle contractile properties, initiating a lengthening of the muscle sarcomere and subsequently increasing actin and myosin proximity (Figure 3). This increased proximity leads to increased cross- formation, which produces higher force production.
​
The Stretch Reflex
The second mechanism of improved force production may be gained through the stretch reflex. Intriguingly, the stretch reflex mechanism may not be produced concurrently in all muscles that are stretched during a plyometric exercise. This is because the muscle response is dependant depends on the number of joints that are crossed and also the activity that is being performed. These differences in reflex muscle activity between mono- and biarticular muscles may be due to the differences in the changes in muscle length during loading. Without muscle fascicle lengthening the muscle spindles may not be stimulated, which may be related to the inconsistent reflex muscle activity in muscles crossing multiple joints during plyometric activities.

Figure 3: Muscle fibre with actin and myosin
The Series Elastic Components
A third mechanism related with the SSC is the storage of potential elastic energy in the series elastic components (actin and myosin filaments within the muscle and the tendon). The series elastic components are stretched when the joint is loaded; however, the tendon has been reported to be the main contributor to length changes in muscle-tendon units and the storage of elastic potential energy.
The Golgi tendon organ (GTO), a sensory receptor that is located in the tendon, is stimulated by the stretch of the tendon. Sensory information from the GTO synapses onto an interneuron in the spinal cord, and inhibitory feedback is sent to the contracting muscle (Figure 4)
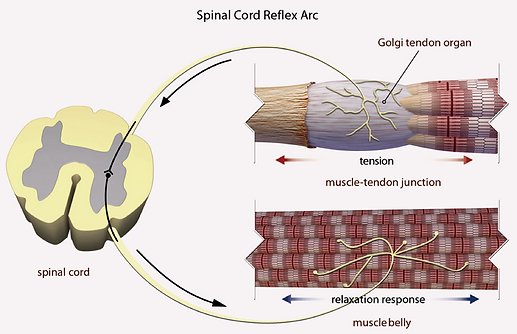
Figure 4: The GTO and its relationship to the neurological system
Previous research suggested that the inhibitory feedback allowed the GTO to function as a means of protecting the muscle from excess tension and thus interfered with forces generated during plyometric exercises (Wilk et al., 1993). Nevertheless, this hypothesis has been challenged by Charmers (2007) due to the ability of the GTO to respond to submaximal forces. Additionally, the excitatory reflexes have been suggested to be initiated by the GTO during movement and may aid in force generation during plyometric activities (Sharman, Cresswell and Riek, 2006). This storage of potential elastic energy can initiate a ‘slingshot’ effect and assist the transfer of positive work that initiates during the coupling phase of the plyometric movement.
​
Coupling Phase
​
The transition between the loading and the unloading phase of a plyometric exercise is often referred to as the coupling phase. This is also commonly referred to as the amortization phase and has also been termed by some as the transmission phase (Horita et al., 2002). For clarity, we shall refer to it as the coupling phase and according to Siff (2004) is the critical phase of the plyometric exercise that determines the effects gained from the SSC. If the transition between the loading and unloading phase is not continuous and quick, the delayed and fragmented coupling will occur and the energy generated will be dissipated and lost as heat.
​
It is important to consider that if there are long delays in the amortization phase the movement will no longer be considered plyometric as the benefits generated during the SSC will be lost. Furthermore, Wilson et al., (1991) suggested that there are measurable decreases in the stored elastic energy that occurs in the coupling phase that lasts over 25-milliseconds. Bosco and colleagues (1981) reported that the average duration of the coupling phase for countermovement jumps is 23-milliseconds and the optimum coupling time may be less than 15-milliseconds. It is important that strength and conditioning coaches should not that training movements that include a delayed amortization phase may have muscle strengthening benefits. However, it is important to note that when implementing plyometric exercises the ultimate goal is to increase power and force production. Stiff (2004) states that athletes should avoid having a noticeable pause in joint movement during the SSC.
​
Unloading Phase
​​​
The final phase of the plyometric movement is termed the unloading phase but it has also been called the rebound, shortening or propulsion phase. The unloading phase of a plyometric movement occurs immediately after the coupling phase and involves shortening of the MTU. Bosco and colleagues (1981) have suggested defined the unloading phase of a single lower extremity joint as beginning when the curve of the joint angle reverses direction and ending when the group reaction force goes to zero. Bobbert and colleagues (1987) suggested that the unloading phase begins at the start of the upward movement of the centre of mass and ends when the toes are no longer in contact with the ground.
​
The unloading phase is often considered as the resultant phase because this part of the plyometric movement is when the mechanisms elicited during the loading phase contribute to increased efficiency of force production. This improved efficiency and force production are gained from the summation of storage and reuse of elastic energy (Kubo et al., 2000), muscle potentiation (Rassier and Herzog, 2005), and the involvement of the myotatic stretch reflex (Gollhofer et al., 1992).
How Plyometric Training Works
​
This section discusses the key aspects that will help to optimise power development through plyometric exercise. The pursuit for optimum power training has steered S and C coaches to develop and use various training methods. Traditionally, heavy resistance training practises have been applied to improve strength and performance. These practices normally involved individuals training using weights of 80 to 95 percent 1RM and performing 1- to 4 repetitions. Contemporary training approaches combine a variety of training modalities incorporating plyometrics and dynamic weightlifting wit the aim of enhancing explosive power.
​
When it comes to athletic programming, the correlation between strength and power is often multifaceted. Predictably, as the resistance increases, the speed of the movement will decline. When individuals perform lifts at maximal effort, the speed of the movement is often slow. Equally, as the overall resistance is reduced, the speed of force development increases, and power will increase. This is because the generation of power is the central factor of success in sport, athletes need to incorporate training techniques that are dedicated on transitioning the strength gained from heavy (but slow) resistance training to high-velocity movements. Plyometric training performed with rapid force absorption and force generation is essential to help the athlete translate strength into power.
​
Contemporary research has suggested that heavy lifting with external resistance and plyometrics as singular approaches of training can successfully improve athletes power output. This has led coaches to believe that by combining both systems the athlete may display greater improvements. This premise has been demonstrated particularly in the area of specific skills such as vertical jumping. The next issue is whether lifting for maximal power output, as opposed to maximum strength, may be of benefit to the athlete.
​
Power Development Using Resistance Exercises
​
For resistance training to be applied successfully to develop power, the lifts must be performed more dynamically. For example the jump squat is a form of squatting that is more fluent. In this type of exercise, the athlete uses a load of circa 30 to 60 percent of 1RM. The exercise involves using all of the fundamental components of muscle contraction that are required to prepare the athlete for higher-intensity plyometric exercises. The goal is to use a resistive load that maximizes the mechanical power output of the exercise.
​
Research has determined that maximal strength levels can be increased by using lighter weights followed by performing highly accelerated movements in both the upper and lower extremities. In the East European literature, this mode of training is termed as a form of complex training. Another form of training involves using heavy weights and quickly freeing yourself from the resistance by dropping or releasing the weight, described as contrast training. Using maximal power training and plyometrics in an integrated manner can lead to increases in power, although the intensity of this mode of training would be overly stressful for long-term training. However, it can be applied for short periods of training, and it is often used in the later stages of the preparation (off-season) cycle of a periodisation training scheme. This type of training can often help to refine the body’s ability to develop force rapidly, resulting in the athlete being able to develop greater impulse forces which are critical in jumping, running, and throwing sports.
​
Contemporary research has been studying the use of the depth jump to improve athletic performance. This maximal-effort plyometric training (also termed shock training) was initially introduced to Russian athletes with the premise that it would help them develop explosive speed and strength. Research on maximal-effort plyometric training has examined drop jumps from heights of up to 3 metres, which pushed the perimeters of safety. This research has also established how the Eastern European athletes were prepared to test the high end of the physical activity intensity continuum to obtain performance gains using plyometric practices. This early research helped to determine that depth jumps were an applicable means of increasing athletes’ speed and strength potential. Athletic training programs by Verkhoshansky’s were founded on resistance training and exercises that included various modes of jumping. The Soviet Olympic feats in track and field ensured that the world took notice. This lead to other coaches and athletes around the world to use similar training and exercises, which became known as plyometrics. Verkhoshansky relied on evidence-based research to make effective training decisions. His manuscripts and books detail¬ his training programs and are respected by elite-level track coaches.
​
Verkhoshansky stated that 0.8m is the optimum height to drop from when looking to attain maximum speed in translating from the eccentric to the concentric phase of the stretch-shortening cycle. He also suggested that 1.1m was the optimum height for developing maximal dynamic strength. Verkhoshansky also recommended no more than 40 jumps be performed in a single workout ad this mode should not be applied more than twice a week. Inter-set Recovery was aided by light jogging and callisthenics exercises. Currently, plyometric training is much less precise in its application and can is amended to allow coaching of each movement skills with submaximal efforts. Additionally, plyometric training is now considered safe for athletic development and also used to reduce injury risk in children and elite performers. A further publication by Verkhoshansky and Tatyan (1983) compared three groups of athletes and demonstrated that depth jumps were more effective than weight training, the jump-and-reach, or horizontal hops for enhancing athletes speed and strength capabilities. Depth jumps are most beneficial in the development of vertical velocity from the ground, which governs how high an individual can jump. Several studies conducted in the United States and Europe since the late 1970s have indicated that depth jumps increase an athlete’s ability to jump higher (vertically) in experimental settings.
​
Contemporary research has endeavoured to examine the differences between training with the depth jump (DJ) versus the countermovement jump (CMJ) Holcomb et al., 1996; Gehri , Kleiner and Kirkendall, 1998). The mechanical difference between these two modalities is that the CMJ is merely flexing the hips, knees, and ankles, allowing for a rapid descent of the body’s centre of gravity before applying concentric muscle activity to jump vertically (Figure 5). This is what is achieved when a person performs a jump-and-reach test. The DJ, on the other hand, involves the use of the individual's body weight to eccentrically load the muscles by a vertical drop from a predetermined height. This movement requires the athlete to time the drop and be psychologically prepared to the inverse (counter) the drop (eccentric to concentric muscle action) at the time the feet makes contact with the ground. Previous data suggest that athletes trained with plyometric exercise such as the DJ, improve both peak power and vertical jump height compared to any other jumping modes.
It is important to note that motor learning plays a significant factor in an athlete’s ability to efficiently use the depth jump in an exercise program. For an athlete to achieve maximum outcomes they must learn and adhere to aspects associated with timing and placement during the program. Available evidence has suggested that the absolute jump heights between DJ and CMJ display a large variance when ground contact time is reduced. This shows the significance of training as specifically as possible for the sport. For example, if the sport needs a jump to be initiated from a static squat position (e.g., basketball block), athletes should train with continued stance times and CMJ practices to optimise the distinct transfer of training. However, if optimum athletic performance involves rapid loading and jumping, the athletes may get the most value from DJ training. Nowadays, the depth jump is not only one of the most examined movements related to sports performance, but it is also frequently used in the assessment of athletes’ risk of injury.

Figure 5: Squat jump [left]; Counter Movement Jump [Centre]; Drop Jump [Right]
Mechanisms of Vertical Jumping
​
Vertical jumping (VJ) is an element of most sports actions. Individuals often take it for granted that an athlete knows how to perform a vertical jump. In reality, jumping vertically is a skill that should be coached by athletes. If we examine VJ movement more carefully, we find that the jump is preceded by a countermovement in which the individuals centre of gravity drops rapidly. This is displayed when an athlete flexes the hips, knees, and ankles of the athlete. The athlete's trunk tilts marginally forward, and their arms are pulled to a position behind the midline of the body (Figure 5).
​
Before the vertical movement of the body, a quick extension of the hips, knees, and ankles takes place, which is the outcome of force generated by the arms and legs. The athlete's arms should be brought forward quickly and allowed to travel to a position above and in front of the shoulders. The quick bend of the knees lowers the centre of gravity of the athlete and is accompanied by the arms moving into a position where the shoulders are extended and the arms are behind the body. This allows the individuals arms to develop force that is directed into the ground as the arms come forward.
​
Importantly, the arms contribute to the generation of force into the ground only whilst they are swinging downward. The force placed into the ground is then recovered as the arms move past the individual's midline and into a position in front of the shoulders. The athlete may envision that they are like a compressed spring and by moving into the squat position with the legs and swinging the arms down quickly the following occurs. The athlete extends the legs and as the arms move past the midline of the body, the pressure on the spring is released. For athletes to generate maximum force they must get their arms as far back and as straight as possible. The more the arms bend at the elbow, the faster they will come through, but the less they will contribute to overall force development. For an applied perspective compare the arm swing of an elite triple jumper with an elite high jumper. Longer levers develop more force; shorter levers move more rapidly. Whereas maximum force is important for the triple jumper, high jumpers using the flop technique must rely more on arm speed to successfully perform this method.
​
Harman et al., (1991) reported that the countermovement is essential in the development of force and that it can contribute as much as six per cent towards the total high jump. Moreover, the arm action increases the overall jump height up to 21 per cent. Harman and colleagues suggested that the arms developed their positive effect by applying a downward force on the body as the swung through in the initial phase of the jump. The arms also ensured that the body positioning was correct where the quadriceps and gluteus muscles could apply force over a longer period. Another inference made was that the countermovement did not contribute significantly to jump height and may not be necessary for many sport situations. If reaction time and speed of movement are more important then the athlete may start the jump with their knees less bent.
​
Optimising Plyometric Training Using Physiology
Founded on the previous examples and outcomes of various investigations, it is evident that refined modifications to plyometric practices can have a significant effect on power output and sport-related performance in tasks that involve the SSC. In most sport skills, eccentric muscle actions are quickly followed by concentric actions. For example, when a long jumper makes contact with the take-off board, their body absorbs the shock of landing by implementing slight flexion of the hip, knee, and ankle. This is followed by a quick extension of the take-off foot and leg as they leave the board (Figure 6).
​
Another example is to a basketball player who looks to perform a slam dunk. As the player takes the last step toward the basket, the supporting leg must take the full body weight and stop the horizontal inertia of the run-up. This loads the leg by quickly forcing the muscles to stretch and undergo a quick eccentric action. Nerve receptors firing information to the muscle then produce a concentric action. These responses transpire with no conscious thought. The amortization phase is critical in distinguishing the regular athlete from the elite athlete. Successful execution of this timing phase is the result of training and motor learning from plyometric exercises. Importantly, all individuals have genetic limitations in speed and power. However, they all have a prospect in maximising their capabilities if coached and trained properly. Even minor differences can lead to significant improvements if they are executed at the correct time.
​
Plyometric actions conclude with a momentum phase, during which the body segments are propelled as a result of the forces summated during the unloading phase (e.g. the flight of a jump) and captured during the coupling phase. The performance outcome achieved during the momentum phase, such as the jump height, is increased compared to the same activity being performed without the SSC. The magnitude of performance development during the momentum phase depends on the degree of the forces and the velocity of the movement during the plyometric activity. Specifically, higher forces are related with a shorter coupling phase and with greater energy stored in the series elastic component. Performance is also a result of the total duration of contact as the contact duration becomes shorter, higher forces and joint moments are generated, and the tendon contribution to work is increased. As mentioned previously, this coupling of the eccentric-concentric action must be quick and begins and ends within hundredths of a second. Typically, elite level high jumpers are on the ground for only 0.12 seconds.