Speed & Agility

Developing Agility
​
For most sports, the ability to rapidly change direction is often the difference between success and failure. In almost all sports athletes require their whole body to accelerate, decelerate, or change direction instantly in response to the sporting conditions. In the real world, most sports quickly altering the athlete’s direction is more important than linear sprint speed. This has resulted in many strength and conditioning coaches seeking effective training modes that will improve their athlete’s agility and acceleration.
​
Young and colleagues (2002) endeavored to identify several key factors that could influence an athlete’s agility and performance. Specifically, they separated agility into two categories; (i) changing directional speed and (ii) perceptual awareness and athlete’s decision-making capabilities with subcomponents as outlined in figure 1. It is important to be aware that agility and quickness are complex sporting skills that include physiological and cognitive mechanisms. An example is the corner kick in football [soccer] where players wait and then immediately decide which method is appropriate to receive or defend the ball. This is a key example of how individuals must move and think quickly as a result of the presented stimulus. Therefore, to maximize athletic performance, training programs need to address both physical and cognitive elements of agility and quickness. If these components are carefully applied then this will help athletes bridge the gap between practice and competition.
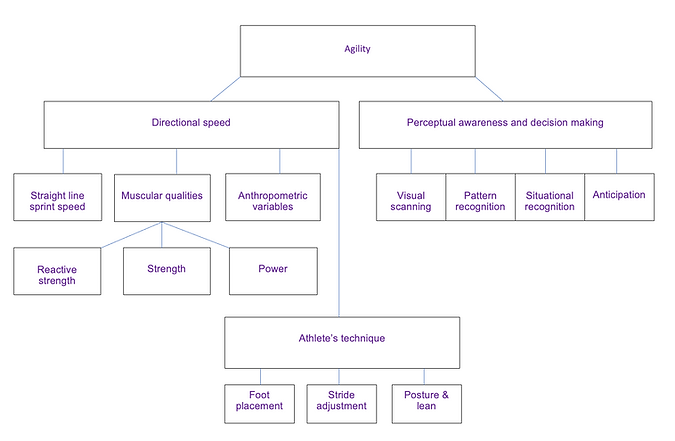
Figure 1. Various elements of agility
Factors Determining Agility
​
Most team sports are characterized by rapid acceleration, deceleration, and direction changes within a nine-meter area. Furthermore, court sports require multidirectional ‘first step quickness’ and directional changes within a four-to-10 meter area. According to numerous coaches, an agility task is a rapid whole-body change of direction or speed in direct response to a given stimulus. Agility can be subdivided into both physical and cognitive abilities. These physical qualities include speed, strength, power, and technique, as well as the leg muscles of the individual.
​
Understanding Speed
​
It is important to consider that if an athlete can move quicker than their opponents, they have an advantage. For example, if an individual can get to the ball quicker than their competitor, they have a direct advantage or opportunity to score a goal in football [soccer]. It is for this reason that athletes and coaches in most sports value speed. Speed is generally measured by using linear sprinting over a specified distance (normally between 30-100 meters [40-to-100 yards]). However, if we consider the distance covered in a typical invasion game, athletes rarely sprint more than 27-meters (30 yards) in a straight line before making some form of directional change. This is unless the athlete is a 100-meter sprinter as their sole focus is on linear speed. On the other hand, since most sports require acceleration from a static or stationary state or when transitioning between movements, linear speed is still an asset that athletes should concentrate on when testing and training for sports.
​
Linear sprinting is a physical skill that most individuals have performed since their second year of life with a certain degree of proficiency. Traditionally, sports coaches believed that linear speed was related to the individual’s genetics and could not be significantly be improved by athletic training. However, evidence has now indicated that the appropriate mode of training can improve running speed even at the elite level. The combination of an individual’s stride rate and stride length primarily determines linear speed. Therefore, by considering these factors an athlete can improve linear speed by increasing stride rate while maintaining stride length, increasing stride length while maintaining stride rate, or doing a combination of both.
​
Most sports (excluding track and field sprinting) involve short-duration sprints and rapid changes of direction, followed by rapid accelerations. Therefore, it would not have little benefit for athletes to focus a large portion of their time training to improve speed capabilities for individuals that would rarely reach maximum speed in competition. It would be more beneficial for these individuals to concentrate on training to accelerate. Acceleration can be defined as the rate of change in velocity and efficiency as possible.
The optimum technique for linear sprinting in the acceleration phase involves four aspects that maximize stride length and stride frequency (Figure 2).
​
-
The individual’s body should have a distinct forward lean that results in a lower center of mass. Therefore, momentum in a linear direction increases. This position also initiates foot contact with the ground under or to some extent behind the center of mass, reducing forces that cause an athlete to slow down or brake.
​​
-
When the athlete pushes off the group during the propulsion phase, the foot touches the ground in a ‘cocked’ position, with the ankle flexed upward at or near 90 degrees and the toes pointed back toward the shin. When the athlete‘s foot contacts the ground the hips, knee, and ankle concurrently with as much force as feasible. This is commonly known as a triple extension.
​​
-
During the recovery phase, the ankle of the free leg should be dorsiflexed while the knee and hip are bent (or flexed). This enables the athlete’s foot to pass directly under the buttocks and a more rapid turnover at the hip.
​​
-
The athlete should make sure that they initiate an arm swing at the shoulder with the elbow flexed at 90 degrees. They should practice swinging the arm forcefully backward to create a stretch reflex that provides more arm forward propulsion.
In the propulsion phase, the power output and rate of force development of the muscles that incorporate the hip extensors and quadriceps muscles contribute to both stride length and stride frequency. In the recovery phase of the linear sprint, the hip flexors and the hamstring muscles are the main contributors to stride frequency. The strength and power of the hip flexors are essential in rotating the hip rapidly from an extended position to a flexed position in preparation for the upcoming foot contact. The hamstrings have an essential role as a multi-joint muscle group because these muscles cross over both the hip and the knee joints and are responsible for decelerating the lower leg during the recovery phase. While this is transpiring, they also immediately transition to assist the hip in extending for the propulsion of sprinting.
This is in contrast to backpedaling when the hamstring muscles are less active and the quadriceps are engaged. Lateral movements involve more activity from the hip abductors (takes away from the body’s midline) than forward sprinting does. It is important that training programs are focused on the development of enhancing agility with particular attention to strengthening the hip flexors, hamstrings, and the muscles that surround the hips. Another factor for consideration that contributes to speed is flexibility. If the hamstrings are disproportionately tight the individual may not be able to bring their knee up as high as possible during the recovery phase of sprinting, limiting hip flexion and speed. Moreover, restrictive hip flexors may limit the ability to extend the hip through the full range of motion, thus reducing power output during the triple-extension phase of propulsion. Correct flexibility of the involved joints contributes to movements that are more dynamic and coordinated, resulting in longer and faster strides and greater speed.
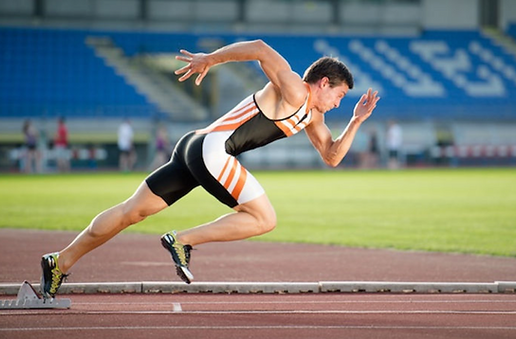
Figure 2. Linear sprinting
What is Strength
Strength is the maximum force that a muscle or muscle group can produce. In most sports, athletes are incapable of reaching their optimum strength levels due to the speed at which they are traveling. Strength is essential, but so is the ability to utilize that strength to generate force. Force is calculated with the following equation:
-
Force = Mass x Acceleration
Therefore, the force can be changed by increasing the mass of the object being moved, increasing the acceleration of a given object’s mass, or with a combination of both. Athletes often increase their mass to improve their force. However, as mass increases (or as weight is gained) athletes must be sure to maintain their ability to accelerate. Adding weight, even if it is lean mass, may not improve performance if it means the athlete will lose a significant amount of speed.
Strength is a key contributor to agility and to athletic performance. When developing the athlete's agility, increasing force to move the body quickly relates directly to strength. Hence, relative strength is more important than absolute strength. The following are the key facets of strength that should be considered when designing a training program for improving agility including concentric, eccentric, and stabilization strength.
​
Understanding Concentric Strength
​
Concentric strength refers to the force exerted by a muscle or group of muscles when it shortens in length. For example, think of performing a biceps curl and bringing the weight upward. Lifting this weight requires a concentric movement of the biceps. Another term to consider is that of positive work in which the force exerted against external resistance results in joint movement in the same direction as the force or in the opposite direction of the external resistance. An example is the push-off during a running, jumping, or cutting movement that is followed by a powerful triple extension of the hip, knee, and ankle. In this and any event, gravity works on the body to pull it down, however, with a powerful extension the individual can overcome the force of gravity and can more efficiently run forward, jump, or make a cutting movement. This ultimately will help them improve their sporting performance levels.
​
Hypothetically, the more force the foot exerts against the ground during running or jumping, the greater the acceleration of the body mass will be. Equally, the greater the force developed by the hip flexors during the recovery phase of running, the greater the forward acceleration from the hip. The increased force from the hip flexors also permits the athlete to position the foot more quickly for contact with the ground. This, therefore, produces greater stride frequency during straight-line sprinting and directional changes. Academic literature suggests that a strong correlation exists between muscular strength and explosive movements, such as both vertical and horizontal jumping, sprinting, and agility movements. The relationship between concentric strength and explosive movements is even more distinct when relative strength is considered. Relative strength factors in the size and weight of an athlete.
​
With absolute strength, if two athletes both squat 120 kg, they have the same maximum lift. If one of the athletes weighs 68 kg and the other weighs 125 kg, the lighter athlete’s relative strength is much greater than their colleague's. The heavier athlete would need to improve relative strength in order to be more explosive. However, the relationship between concentric strength and explosive movements becomes less obvious when studying elite-level athletes. Contemporary evidence suggests that there is a threshold in strength at which further improvements in explosive movement performance are more associated with the rate of force development. Maximum concentric strength is particularly important in the acceleration phase of sprinting. Since acceleration is an integral aspect of agility technique, the role of concentric strength in maximizing agility performance is essential.
​
Eccentric Strength
Eccentric strength refers to the force exerted by a muscle or groups of muscles as they lengthen. For example, think of lowering the weight back to the starting position during a biceps curl. Another term frequently used is negative work in which the force exerted against external resistance results in joint movement in the opposite direction of the force or in the same direction as the external resistance. An athlete with high eccentric strength can rapidly and efficiently decelerate their body while maintaining a dynamic balance in preparation for a change in direction. The ability to decelerate the body quickly while maintaining control is another important contributor to movements that involve rapid directional changes. Impaired eccentric strength can reduce deceleration and limit the athlete's ability to change direction quickly.
​
The correlation between eccentric strength and the ability to decelerate is demonstrated by the movements in a stretch-shortening cycle (see the plyometric page). To limit contact time with the ground during a stretch-shortening cycle, suitable eccentric strength is fundamental for decelerating the body mass quickly so it can be accelerated in a new direction. The individual's ability to decelerate is important for both performance and injury prevention. Athletes can attain the greatest amount of force during eccentric muscle action. Most injuries occur during joint deceleration. One of the central contributors to correct deceleration is the eccentric strength of the involved musculature. If these structures are not strong enough to withstand forces during movement, poor body mechanics can lead to improper body position, increasing the injury risk. However, resistance and plyometric training of the eccentric strength allows athletes to increase their ability to decelerate body mass. This can translate into improved agility and athletic performance.
​
Stabilization Strength
​
Joint stability is an essential but often disregarded aspect that contributes to the effective application of force during agility movements. Agility training requires strengthening the muscles involved in stabilizing the trunk (see core strength and posture) and the joints of the lower extremities. For example, when the athlete's foot touches the ground during a plant-and-cut movement, forces from the ground are transmitted up through the legs, hips, and trunk. If the musculature adjoining these joints and supporting the trunk is not stabilized by muscular contraction, then too much force may be absorbed or lost at these sites, impairing the transition from eccentric to concentric movements.
This, therefore, results in inefficient movements and suboptimal skill performance. An example of this is an athlete running a multi-directional cone-agility drill. If due to inadequate core stability, the athlete lacks the capability to decelerate lateral forces when performing a cutting motion, they will take longer to make a directional change. This theoretically increases the athlete’s risk of injury. If a similar athlete who has the ability to stabilize the body and efficiently change directions were to perform this same action, they may experience greater success and fewer injuries due to their movement proficiency.
The strength that enhances stabilization is also essential for muscle balance. For example, during hip extension in the push-off portion of sprinting, the gluteus maximus must contract to create the explosive movement that thrusts the body forward. However, the gluteus maximus also helps rotate the hip outward. Lack of control of this unnecessary movement hinders the athletes’ ability to propel themselves forward. To resist undesirable movement at the hip, the adductor magnus must contract to improve the stability of the hip joint. This ensures that the force created by the gluteus maximus is used for the forward momentum of the body and is not used on other movements. In addition, the medial hamstrings and the lateral gastrocnemius muscles aid in controlling undesirable movement at the knee joint during cutting manoeuvres. Both enhance the performance of these movements and reduce the risk of injury.
Resistance-training exercises can improve the strength and timing of the muscles’ stabilizing contributions, including both bilateral and unilateral drills, such as the following:
-
Multi-joint movements, such as the back squat and forward, backward, and diagonal lunges.
​​
-
Single-limb training, such as single-leg squats and other single-leg movements.
​​
-
Explosive plyometric movements were performed with the correct technique, such as single-leg bounding and single-leg hops.
Intermuscular coordination is another vital feature of muscular contraction that is closely related to stability during movement. Each muscle can transmit signals and information to other muscles in the system. The speed at which they communicate relates to the activation timing of various muscles across a joint. Intermuscular coordination is important for running speed because if the hamstrings are not relaxed when the thigh is brought forward in the recovery phase of the stride, hip flexion will be reduced, resulting in a shorter stride length. This is particularly true in movements involving directional changes, where joint stability is of greater concern for the athlete. For example, football (soccer) players who are more experienced exhibit more coordinated patterns of muscle activation during cutting movements than less-experienced individuals do. Training involving acceleration, deceleration, and directional changes suggests contributing to improved intermuscular coordination and, in turn, better agility performance and a lower risk of injury.
Intramuscular coordination relates to an individual muscle’s ability to improve motor-unit recruitment, rate coding, and motor-unit synchronization. The greater the number of motor units an athlete can recruit at a given time, the greater his ability to produce force. Equally, with rate coding, as the intensity of stimulus increases, the rate of peak firing also increases. When these units are recruited quickly and in the appropriate sequence, an athlete can express this force over a reduced period of time, improving the overall potential for speed.